Filter Results
Related Organization
- Biological and Environmental Systems Science Directorate (23)
- Computing and Computational Sciences Directorate (35)
- Energy Science and Technology Directorate (217)
- Fusion and Fission Energy and Science Directorate (21)
- Information Technology Services Directorate (2)
- Isotope Science and Enrichment Directorate (6)
- National Security Sciences Directorate (17)
- Neutron Sciences Directorate (11)
- Physical Sciences Directorate (128)
- User Facilities (27)
Researcher
- Chris Tyler
- Justin West
- Ritin Mathews
- Brian Post
- Blane Fillingim
- David Olvera Trejo
- J.R. R Matheson
- Jaydeep Karandikar
- Lauren Heinrich
- Peeyush Nandwana
- Scott Smith
- Sudarsanam Babu
- Thomas Feldhausen
- Yousub Lee
- Akash Jag Prasad
- Alexander I Wiechert
- Alex Roschli
- Brian Gibson
- Calen Kimmell
- Costas Tsouris
- Debangshu Mukherjee
- Emma Betters
- Erin Webb
- Evin Carter
- Greg Corson
- Gs Jung
- Gyoung Gug Jang
- Jeremy Malmstead
- Jesse Heineman
- John Potter
- Josh B Harbin
- Kitty K Mccracken
- Md Inzamam Ul Haque
- Mengdawn Cheng
- Olga S Ovchinnikova
- Oluwafemi Oyedeji
- Paula Cable-Dunlap
- Radu Custelcean
- Ramanan Sankaran
- Soydan Ozcan
- Tony L Schmitz
- Tyler Smith
- Vimal Ramanuj
- Vladimir Orlyanchik
- Wenjun Ge
- Xianhui Zhao
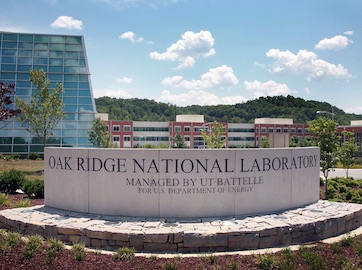
System and method for part porosity monitoring of additively manufactured components using machining
In additive manufacturing, choice of process parameters for a given material and geometry can result in porosities in the build volume, which can result in scrap.
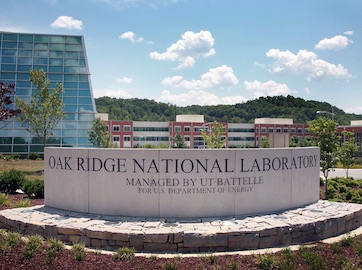
Among the methods for point source carbon capture, the absorption of CO2 using aqueous amines (namely MEA) from the post-combustion gas stream is currently considered the most promising.
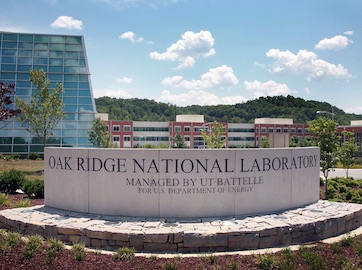
Distortion generated during additive manufacturing of metallic components affect the build as well as the baseplate geometries. These distortions are significant enough to disqualify components for functional purposes.
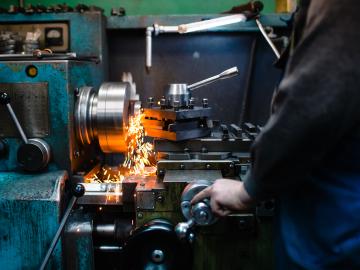
For additive manufacturing of large-scale parts, significant distortion can result from residual stresses during deposition and cooling. This can result in part scraps if the final part geometry is not contained in the additively manufactured preform.
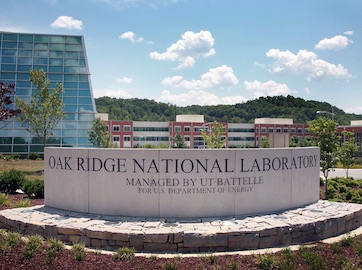
The use of biomass fiber reinforcement for polymer composite applications, like those in buildings or automotive, has expanded rapidly due to the low cost, high stiffness, and inherent renewability of these materials. Biomass are commonly disposed of as waste.
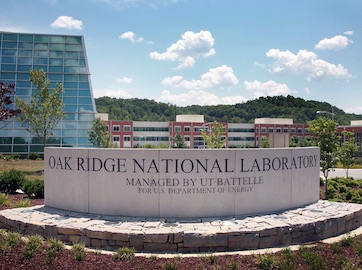
In additive manufacturing large stresses are induced in the build plate and part interface. A result of these stresses are deformations in the build plate and final component.
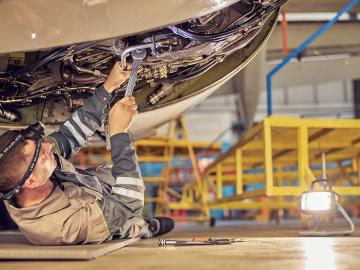
Materials produced via additive manufacturing, or 3D printing, can experience significant residual stress, distortion and cracking, negatively impacting the manufacturing process.
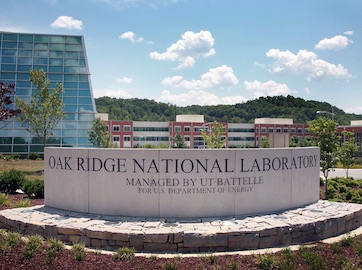
This work seeks to alter the interface condition through thermal history modification, deposition energy density, and interface surface preparation to prevent interface cracking.
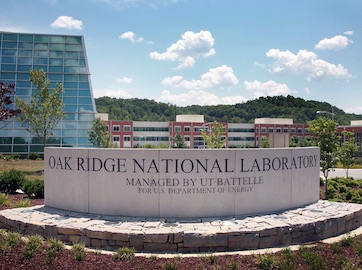
Additive manufacturing (AM) enables the incremental buildup of monolithic components with a variety of materials, and material deposition locations.