Achievement: Computational scientists and neutron structural biologists from Oak Ridge National Laboratory developed an integrated workflow using small-angle neutron scattering (SANS), atomistic molecular dynamics (MD) simulation, and an autoencoder-based deep learning (DL) algorithm to characterize structural ensembles of intrinsically disordered proteins (IDPs). IDPs make up 33% to 50% of eukaryotic proteomes and are involved in critical cellular function and regulation. However, IDPs lack well-defined three-dimensional structures which makes them challenging to probe experimentally and computationally. Here, the team performed SANS experiments that used both full contrast and contrast matching with residue-specific deuterium labeling to gain insights into structural dynamics of an IDP complex. They generated individual IDP complex structures with microsecond atomistic MD simulations and observed transient helix and loop conversion in these structures. Such subtle structural changes affect the protein complex interface and are important for IDP function. However, these structural changes are indistinguishable by conventional depictions of structural ensembles. The developed DL algorithm successfully discerns these different structures in the latent space.
Significance and Impact: Their approach has made an impact on both experiment and computation for studying IDP structural ensembles. The residue-specific deuterium labeling of SANS experiments is innovative and has not been demonstrated in IDPs before. In addition, integration of MD and DL complements conventional structural analysis techniques and enables comprehensive comparison of high-dimensional protein structures without prior knowledge of the protein systems, which is particularly challenging for highly flexible proteins such as IDPs.
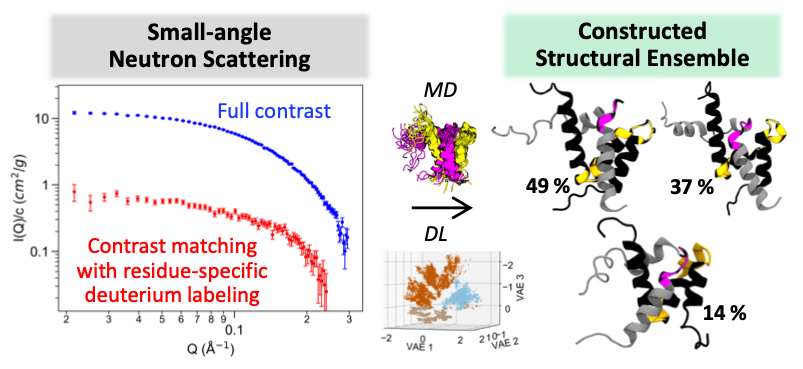
Research Details
- Performed SANS experiments that used both full contrast and contrast matching with residue-specific deuterium labeling.
- Performed 12-microsecond atomistic MD simulations with four molecular mechanics force fields.
- Compared MD structural ensembles with NMR experiments from the literature and in-house SANS experiments using chemical shift, neutron scattering intensity, and radius of gyration.
- Characterized MD structural ensembles by helical fraction, average C RMSF, all-atom RMSD with respect to an initial NMR structure, potential of mean force, and DL convolutional variational autoencoders.
Facility: SANS experiments were performed at SNS; MD simulations and DL analysis were performed at CADES.
Sponsor/Funding: DOE ASCR
PI and affiliation: Serena Chen (CSED)
Team: Kevin Weiss (NSD), Chris Stanley (CSED), Debsindhu Bhowmik (CSED)
Citation and DOI:
Chen, SH, Weiss, KL, Stanley, C, Bhowmik, D. Structural characterization of an intrinsically disordered protein complex using integrated small-angle neutron scattering and computing. Protein Science. 2023; 32(10):e4772. https://doi.org/10.1002/pro.4772
Summary: This study presents an integrated SANS, MD, and DL workflow to effectively distinguish different protein conformations and characterize challenging structural ensembles of IDPs, such as the NCBD/ACTR complex. The NCBD/ACTR complex is the first example of coupled binding and folding of IDPs and has a long-known association with breast and ovarian cancers. Although the complex is more ordered than the unbound proteins, about 20% of their residues remain highly flexible in the complex, which motivates our study to characterize its structural ensemble. Based on the known NMR structures, the transiently formed helices of the two proteins form the interaction interface. However, it is not known how these transient helices behave in dynamics and how structural changes may affect the interface of the protein complex. Therefore, we performed atomistic MD simulations and SANS experiments and compared the MD structural ensembles with NMR experiments from the literature and our SANS data. The SANS experiments of both full contrast and contrast matching with residue-specific deuterium labeling provide experimental structural metrics such as the scattering intensity I(Q) curves and radius of gyration Rg for analyzing our MD structures. Based on the MD structures selected from the constructed free energy surface, we observed conversion between helices and disordered coils. Such transient secondary structure changes affect the shape of the accommodating groove formed by helices 1 and 3 of NCBD and affect the docked conformation of ACTR. However, these subtle global and local structure changes are undetectable on the free energy surface and only become apparent when visualizing their three-dimensional structures. We therefore sought another approach to compare these structures more effectively. To this end, we developed a DL algorithm to complement potential of mean force calculations and enable comprehensive comparison of high-dimensional molecular structures of the NCBD/ACTR complex.
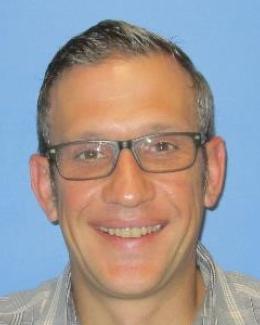