Project Details
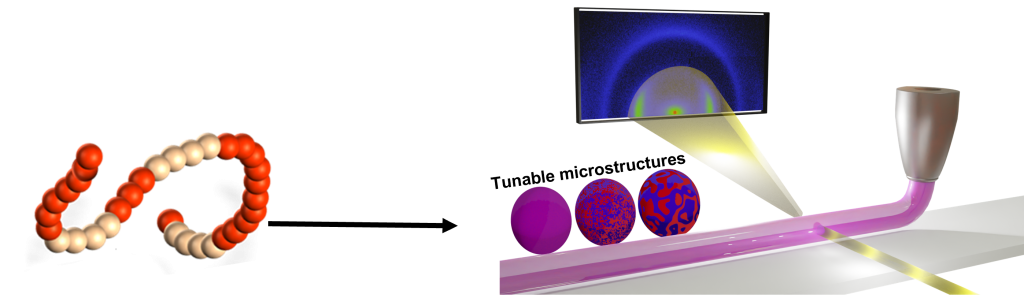
The overarching goal of this proposal is to gain a fundamental understanding of how the complex physical and chemical interactions between highly branched, network-forming multi-dimensional macromolecules can be tuned to control bulk properties in additive manufacturing. A challenge with additive manufacturing (AM) using traditional linear polymers is that layer-by-layer deposition traps nonequilibrium states producing residual stresses that impede interdigitation and bonding between the layers resulting in poor mechanical performance. Currently, there are no guidelines for the a priori design of polymer building blocks for high throughput AM that directly address these issues. Thus, there is a critical need for fundamental insight into how the polymer molecular architecture can be tuned to provide the desired properties for AM which involves non-equilibrium states and dynamics to improve the performance of polymeric parts manufactured by AM. Our central hypothesis is that branched multi-dimensional polymers can be used to regulate the relaxation dynamics and drive the intended formation of metastable states that provide enhanced material properties and enable process circularity in AM. Specifically, it is proposed that polymers containing rigidizing, long-chain backbones and branches decorated with shorter fast-relaxing chains end-capped with reversible covalent bond-forming moieties will enhance interdigitation and bonding between layers and provide the desired mechanical properties. To test this hypothesis, we will synthesize and characterize model branched polymers containing molecularly heterogeneous phases to circumvent the existing paradigm of tradeoffs between stiffness, toughness, and processability in AM. The inclusion of reversible covalent networks, secondary to key physical interactions and entanglement, can enable chemical and physical consolidation at multiple length scales for energy-efficient manufacturing as well as conversion back to feedstock and remanufacturing. This proposal will be organized around the following research aims, each addressing critical scientific questions:
Aim 1 – Understand how the chemistry and structure of branched copolymers provide new pathways to control polymer interactions and properties: Can branch density and chemistry control fundamental polymer dynamics and their relation to bulk properties? Can we controllably increase the branch density to deliver high molecular weight multidimensional macromolecules with superior properties? Aim 2 – Understand how reversible secondary network formation in highly branched polymers impacts the properties of multidimensional materials for circular manufacturing: Can we control multiphase structures, dynamics, and properties in branched macromolecules via secondary network formation? Can we switch between metastable and equilibrium states using reversible networks and design pathways to (re)-manufacture multidimensional printed structures? Crosscut Aim – Elucidate structure-property processing relationships through operando characterization and modeling for new soft matter designed for AM: Can we capture the key underlying physical and chemical events in AM by operando spectroscopy, scattering and modeling?
An interdisciplinary team will combine multi-scale modeling alongside x-ray and neutron scattering, spectroscopy, and nanoscale imaging techniques to understand the dynamic processes to design and model new materials specifically for AM. The proposed research addresses multiple priority research directions (PRDs) outlined in the Basic Research Needs (BRN) Reports: Transformative Manufacturing―PRD#3 and PRD#5 and BRN for Synthesis Science―PRD#3. This research will establish how macromolecular architecture and interfacial structure-properties-interactions affect macroscopic properties needed for materials and energy efficiency in additive manufacturing for enhanced manufacturing competitiveness.
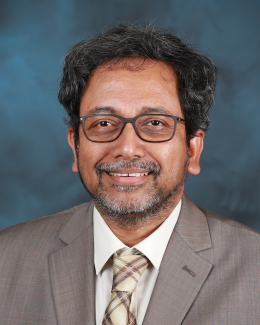