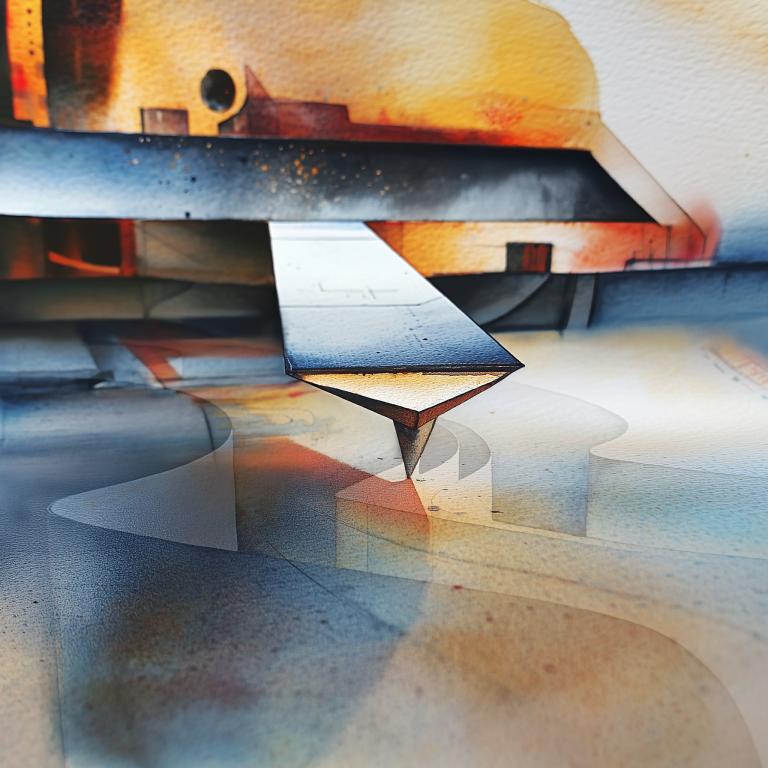
As demand for energy-intensive computing grows, researchers at the Department of Energy’s Oak Ridge National Laboratory have developed a new technique that lets scientists see — in unprecedented detail — how interfaces move in promising materials for computing and other applications. The method, now available to users at the Center for Nanophase Materials Sciences at ORNL, could help design dramatically more energy-efficient technologies.
Data centers today consume as much energy as small cities, and that usage is skyrocketing. To counter the trend, scientists are studying exotic materials such as ferroelectrics that could store and process information far more efficiently than silicon, which is traditionally used. But realizing the potential depends on understanding the processes occurring at dimensions thousands of times smaller than a human hair — specifically, at the ferroelectric material’s domain walls, which are the boundaries between areas of the material that exhibit different magnetic or electric properties.
“Domain walls can have completely different properties from the neighboring domains they separate,” said ORNL’s Neus Domingo. “Some might conduct electricity — despite the bulk material being nonconductive — while others show magnetic behavior despite the surrounding material being nonmagnetic. These differences matter because they may enable us to use them as new nanoelectronic components to store and process signals at the tiniest scales, which is key for developing next-generation low-power devices such as memory chips and sensors.”
Researchers unveiled a new technique that captures detailed views of how domain walls move under rapidly fluctuating electric fields. As described in the journal Small Methods, the technique, called scanning oscillator piezoresponse force microscopy, reveals both slow and sudden movements in these boundaries, providing new insights into energy management at extremely small scales.
The method fills in crucial gaps by creating dynamic visualizations that allow scientists to observe how domain walls move and better estimate how much energy is required to shift them. It turns a static snapshot into a vivid, explanatory sequence.
Understanding and controlling the behavior of such domain walls without this new technique is like trying to coach a football game from just two still shots: one showing the quarterback lined up before the snap, and another capturing the moment after the play has ended. Without knowing the events in between, it is much more difficult to know whether the play was a run or a pass, which players were critical or what effort led to the result. Improving how the team plays requires being able to see all the action.
“The method fills in crucial gaps by creating dynamic visualizations that allow scientists to observe how domain walls move and better estimate how much energy is required to shift them. It turns a static snapshot into a vivid, explanatory sequence,” said ORNL’s Stephen Jesse. “Using precisely timed measurement and control electronics, we can rapidly and systematically change the state of a ferroelectric material and watch how changes evolve over time. Until now, this level of detail has not been achieved using atomic force microscopy, and the method can be adapted for use in electron microscopes and other advanced instruments.”
The technique captures both the tiny, repeated movement of domain walls as they react to changes in their environment — such as electric or mechanical signals — and the sudden, stop-and-go movements that happen when the walls are briefly stuck and then abruptly shift, like a door sticking before it swings open.
These patterns of motion, both smooth and jerky, reveal how ferroelectric polarization is modified within the material. Ferroelectric polarization is the natural alignment of electric charges in certain materials that can be changed by an external electric field, and this ability is crucial for applications such as memory storage and sensors.
The researchers aim to further refine this technique to study other materials and collaborate with industry partners to explore potential commercial applications.
The DOE Basic Energy Sciences program funded this research. The experiments were performed at the Center for Nanophase Materials Sciences, a DOE Office of Science user facility at ORNL.
UT-Battelle manages ORNL for DOE’s Office of Science. The single largest supporter of basic research in the physical sciences in the United States, the Office of Science is working to address some of the most pressing challenges of our time. For more information, please visit energy.gov/science. — Scott Gibson