March 27, 2018 – Scientists at the Department of Energy’s Oak Ridge National Laboratory are conducting fundamental physics research that will lead to more control over mercurial quantum systems and materials. Their studies will enable advancements in quantum computing, sensing, simulation, and materials development.
The researchers’ experimental results were recently published in Physical Review B Rapid Communication and Optics Letters.
Quantum information is considered fragile because it can be lost when the system in which it is encoded interacts with its environment, a process called dissipation. Scientists with ORNL’s Computing and Computational Sciences and Physical Sciences directorates and Vanderbilt University have collaborated to develop methods that will help them control—or drive—the “leaky,” dissipative behavior inherent in quantum systems.
“Our goal is to develop experimental platforms that allow us to probe and control quantum coherent dynamics in materials,” said Benjamin Lawrie, a research scientist in the Quantum Sensing Team in ORNL’s Quantum Information Science Group. “To do that, you often have to be able to understand what’s going on at the nanoscale.”
Bringing perspectives from quantum information science, nanoscience and electron microscopy, the scientists exploit existing knowledge of matter and the physics of light and sound to examine the quantum nature of nanostructures—structures that measure about one-billionth of a meter.
One project focused on driving nitrogen vacancy center defects in nanodiamonds with plasmons. The naturally occurring defects are created when a nitrogen atom forms in place of the typical carbon atom, adjacent to an atomless vacancy. The defects are being investigated for use in tests of entanglement, a state that will allow substantially more information to be encoded in a quantum system than can be accomplished with classical computing.
Electrons generate an electric field. When an electron beam is applied to a material, the material’s electrons are spurred to motion—a state called excitation—creating a magnetic field that can then be detected as light. Working with plasmons, electron excitations that couple easily with light, allows scientists to examine electromagnetic fields at the nanoscale.
Matthew Feldman, a Vanderbilt University graduate student conducting doctoral research at ORNL through the National Defense Science and Engineering Graduate Fellowship program and a member of the Quantum Sensing Team, used a high-energy electron beam to excite nitrogen vacancy centers in diamond nanoparticles, causing them to emit light. He then used a cathodoluminescence microscope owned by ORNL’s Materials Science and Technology Division, which measures the visible-spectrum luminescence in irradiated materials, to collect the emitted photons and characterize high-speed interactions among nitrogen vacancy centers, plasmons and vibrations within the nanodiamond.
In other research, Jordan Hachtel, a postdoctoral fellow with ORNL’s Center for Nanophase Materials Sciences, used the cathodoluminescence microscope to excite plasmons in gold nanospirals. He explored how the geometry of the spirals could be harnessed to focus energy in nanoscale systems. Andy Lupini served the project as a microscopy consultant, providing expertise regarding equipment optimization and troubleshooting.
Precise control over nanoscale energy transfer is required to enable long-lived entanglement in a model explored by Eugene Dumitrescu, a research scientist in ORNL’s Quantum Information Science Group. Dumitrescu’s research, published in Physical Review A in late 2017, showed that the photon statistics Feldman collected could be used in calculations to show entanglement.
“This work advances our knowledge of how to control light–matter interactions, providing experimental proof of a phenomenon that had previously been described by simulations,” Lawrie said.
Closed systems, in which quantum information can be kept away from its surroundings, theoretically can prevent dissipation, but real-world quantum systems are open to numerous influences that result in information leakage.
“The elephant in the room in discussions of quantum systems is decoherence,” Feldman said. “If we can model an environment to influence how a quantum system works, we can enable entanglement.”
Dumitrescu agreed. “We know quantum systems will be leaky. One remedy is to drive them,” he said. “The driving mechanisms we’re exploring cancel out the effects of dissipation.”
Dumitrescu used the analogy of a musical instrument to explain the researchers’ attempts to control quantum systems. “If you pluck a violin string, you get the sound, but it begins to dissipate through the environment, the air,” he said. “But if you slowly draw the bow across the string, you get a more stable, longer-lasting sound. You’ve brought control to the system.”
Feldman thinks these are fascinating times for quantum physicists because the field of quantum computing is at the same phase classical computing was in the mid-20th century. “What excites me most is how current research could change our understanding of quantum systems and materials,” he said.
This research is supported by ORNL’s Laboratory Directed Research and Development program and DOE’s Office of Science. The Center for Nanophase Materials Sciences is a DOE Office of Science User Facility.
ORNL is managed by UT–Battelle for DOE’s Office of Science, the single largest supporter of basic research in the physical sciences in the United States. DOE’s Office of Science is working to address some of the most pressing challenges of our time. For more information, please visit http://energy.gov/science/. — by Laurie Varma
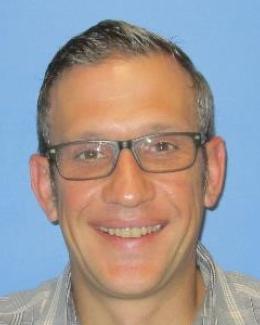