The U.S. economy went through nearly 36 million tons of plastic in 2018, the last year for which we have data. Of that amount, 9 percent was recycled, 16 percent was burned for energy, and the remainder — 30 million tons, or fully three-quarters of the total — ended up in landfills or in the ocean, where it is destined to stay in its current form essentially forever.
Plastics may be our most troubling example of a linear economy, one in which natural resources — in this case, petroleum, for the most part — are used once and then discarded.
But they are not the only example. The Environmental Protection Agency reports that in that same year, two-thirds of cans and other aluminum, 61 percent of bottles and other glass, and 54 percent of discarded tires, shoes and other rubber and leather items also found their way into landfills.
This level of waste is unsustainable, both in terms of the resources being lost and the pollution being created. In order to achieve a sustainable economy, we must be able to take our leftover products and appliances — even our water — and put them to good use again. This is known as a circular economy.
ORNL researchers are deeply involved in applying the principles of a circular economy, focusing, for instance, on plastics and polymers, water and critical materials.
Plastics are forever
One challenge we face in creating a circular economy for plastics is that new plastics are cheaper than their recycled counterparts. Another is that traditional plastics are not designed to decompose.
“Here’s the problem: Most of the plastic that has been produced is still around,” said Rigoberto Advincula, who leads the Macromolecular Nanoscience Group at ORNL’s Center for Nanophase Materials Sciences and serves as Governor’s Chair of Advanced and Nanostructured Materials at ORNL and the University of Tennessee.
“A lot of that goes into landfills, some goes to incineration — where it is burned for energy content — and then the rest ends up in the ocean,” Advincula explained.
The challenge, then, is twofold: first, to find a way to recycle existing plastics and polymers and keep them out of landfills and, second, to create new plastics and polymers that are designed to be easily recycled and reused.
One big challenge to plastics recycling is that plastic waste doesn’t refer to just one material. There are a variety of plastics, each with unique properties. To create valuable products from recycled plastic, then, we must handle the diversity of materials going into the process. This involves breaking them down into their component molecules.
“We have a term called construction–deconstruction chemistry,” Advincula said. “We take these existing polymers, which are large molecules, chop them and start connecting them back.
“Deconstruction–construction means that we need to discover new types of catalysts or reaction mechanisms that allow us to deconstruct them properly and to reconstruct them and then to process them in the melt.”
Putting those component parts back together into economically viable products is a monumental scientific challenge. For Advincula, the best way to make the process successful is to create products that are more valuable than the original plastics. This approach is called upcycling.
“It could be a more high-performance polymer or plastic product,” he said, “or it could be an intermediate for another chemical reaction. It has to have an added value for people to buy and use it.”
Enabling autonomous materials research
Both challenges, then — designing more recyclable materials and recycling those we already have — require a massive amount of experimentation. One approach being taken at ORNL to accelerate the process is the creation of a reconfigurable continuous flow reactor, which is essentially a (nonnuclear reactor) device to automate large-scale chemistry experiments.
The reactor — a three-year investment by ORNL — takes advantage of the lab’s strengths in supercomputing, neutron science and materials science. Planned for the lab’s Center for Nanophase Materials Sciences, the reactor will combine analytical tools such as nuclear magnetic resonance, various forms of spectroscopy and chromatography, X-ray analysis and neutron analysis with artificial intelligence and machine learning to automatically conduct, analyze and rework experiments to recycle existing materials and create new ones.
“In our standard approach, we typically make some material, then we characterize the material, then we try to understand what’s going on in the material,” said ORNL Corporate Fellow Bobby Sumpter, who serves as CNMS’s Theory and Computation section head. “What we want to do here is to do it all at once in a single experiment. And that’s where the AI and machine learning come in, because the number of control parameters you have, and the number of possible compositions you have to deal with, is basically infinite.”
By automating the “make–measure–model” approach of traditional chemistry and using AI to provide feedback to allow autonomous decisions, the reactor can greatly accelerate the discovery process. And while it will be used on a wide variety of important scientific problems, two of these will be the recycling of existing mixed plastics and the creation of smarter future plastics.
“We have focused efforts to use AI to mimic a real chemist or materials scientist in making decisions on which compositions or which catalysts or which reactor parameters are required to deliver a particular material property or a recyclability possibility,” Sumpter said. “Oak Ridge is putting forth a pretty significant investment to build capabilities to not just automate materials synthesis, but to make it autonomous.”
High-tech materials
The challenge of plastics doesn’t just apply to consumer products such as water bottles and grocery bags. It also extends to the high-tech composite materials that go into high-performance plastic products such as electric vehicles, aircraft and wind turbine blades.
By combining plastics with carbon or glass fibers, these composites combine the light weight and strength needed in these 21st century products. Yet, once the cars or windmill blades reach the ends of their useful lifetimes — typically 20 years for a wind turbine blade — the problem of the linear versus circular economy remains. On top of that, high-tech composite materials have a significantly larger carbon footprint than lower-tech plastics, and demand for these composites is expected to grow about 7 to 10 percent each year for the next decade.
According to ORNL materials scientist Soydan Ozcan, who also leads the Institute for Advanced Manufacturing Composite Innovation’s composite recycling effort, the challenge of composites, like that of conventional plastics, requires both short- and long-term approaches.
“We cannot design everything in the next five years to switch to a new world,” Ozcan said. “We’ll have this problem for at least the next 30 years. It’s going to take time to switch to more sustainable material and product designs. In the meantime, we’ve got to develop advanced recycling technologies that will work with current materials.”
Ozcan suggested a variety of uses for these materials. For example, windmill turbine blades can find a second lifetime as structural reinforcements in bridges, buildings and even power poles. The materials can also be broken down and used in advanced 3D-printing applications, a process being demonstrated at ORNL’s Manufacturing Demonstration Facility.
One advantage to recycling in the composites industry is that its waste streams aren’t as contaminated as commodity plastics. However, it is still critically important to minimize contamination in recycled composites to ensure they can be used in the highest-value applications. As such, the material must be tracked throughout its life cycle with techniques such as radio-frequency identification, or RFID, and cloud-based data management.
“We need to capture value throughout the digital supply chain,” Ozcan said. “Where are these wind turbine blades? Where are these cars? Or where are these composite materials? How many times have they been used? How many years have they been used? What type of thermosets or thermoplastics were used? Where are the closest recycling centers or facilities?”
The goal is to keep composite materials out of landfills, take advantage of the energy and cost that has gone into them, and maximize their useful lifetimes.
For example, Ozcan said, "There are many steps in the production of a blade. Raw materials are mined, polymer is then synthesized, fiber is made, and the composite is then manufactured. Each step involves transportation costs — capital, energy and CO2 — before you get the final product and the utility. In 20 years when you are done, you don’t need to redo every step. The structure is still fine and, if designed appropriately, can potentially be used for other purposes.”
Efforts to recycle existing materials are necessarily a stopgap effort as researchers design more inherently recyclable materials, he noted. After all, the materials themselves were not created to be recycled.
“We design for performance, mechanical strength, stiffness, rigidity, longevity and so on,” Ozcan said. “This is typically what I do as a materials engineer. While we were creating these materials, we didn’t talk about what was going to be happening to them at the end of life.
“Composite materials are extremely complex. It’s not easy to unzip and reuse the resin system. So, the starting point should be how we can develop and implement inherently recyclable resin systems.”
Composites may also lend themselves to bio-based materials, he said, allowing manufacturers to forgo petroleum-based materials altogether.
“Instead of starting with a petroleum base, what if we start with biologically sourced materials? When you make a resin or fiber out of wood, your starting material already has a negative carbon footprint because trees and plants process CO2 during their lifetime.”
For Ozcan, ORNL is an ideal place to answer these questions.
“Oak Ridge is a science laboratory. We have an interdisciplinary team of chemists, materials scientists, product design engineers, computational experts and others who can support all of the diverse, complex and necessary aspects of the technology development.”
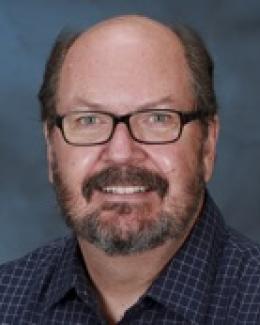