More than 1800 years ago, Chinese astronomers puzzled over the sudden appearance of a bright “guest star” in the sky, unaware that they were witnessing the cosmic forge of a supernova, an event repeated countless times scattered across the universe.
Today, with more than a millennium of observations and a significantly more powerful toolkit, Earth-bound researchers use some of the most advanced computers in the world to piece together the internal mechanisms of these celestial phenomena and the role they play in the creation of the elements.
“The fundamental problem with astrophysics, compared to other fields of physics, is we don’t get to design our experiments,” said Raph Hix, a computational astrophysicist at the Department of Energy’s Oak Ridge National Laboratory. “Mother Nature periodically conducts an experiment in our view, and we race to collect whatever information we can.”
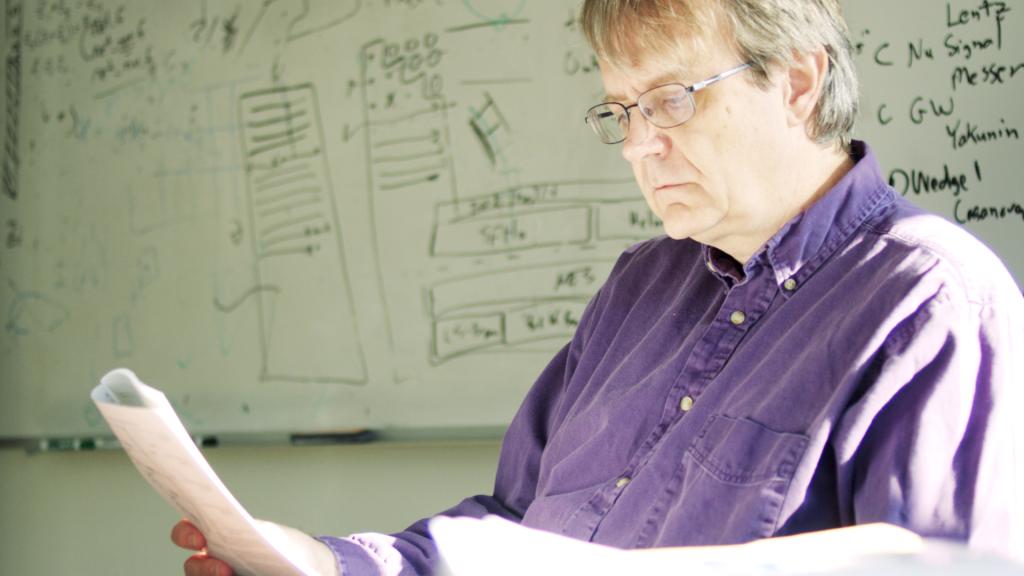
Hix and other ORNL astrophysicists construct models using state-of-the-art supercomputers to simulate the collapse and explosion of massive stars more than ten times the mass of our sun and understand how supernovae create new chemical elements through a process known as nucleosynthesis.
“The job of modeling, especially in astrophysics, is to build a model that matches the observations, then we get to do a deep dive inside and look at the details of the event that are impossible to see because the explosion is happening a megaparsec away and we’re stuck in one location,” Hix said.
To help overcome the limited perception imposed by vast distances, astrophysicists rely on a multitude of observational and experimental data to compare to these multidimensional models of a supernova’s physics. The observations incorporate photometry and spectra collected from optical, ultraviolet, X-ray and gamma ray telescopes, and even include data on isotopic signatures gained from the study of bits of stardust extracted from meteorites, known as presolar grains, created in explosions before the formation of our solar system. By comparing models against this myriad of observations, Hix and his colleagues are able to explore the nucleosynthesis process and how supernovae helped create the heavy elements and isotopes around us.
“At the end of the day, I always come back to the formation of the elements. Understanding how that happens is irrationally fascinating to me,” Hix said.
This fascination began during his time at the University of Maryland, where he majored in physics, astronomy and math. Before lecture one day, Hix started reading about the origin of elements and became enraptured with how they were formed and made their way across the cosmos.
“The oxygen you’re breathing right now came to us because a star 15 or 20 times the mass of the sun blew itself to bits,” he said. “It’s a very personal connection to the universe.”
Hix has been hooked ever since, and his interest in nucleosynthesis carried on through his graduate work at Harvard and his time as a postdoc in Texas before joining ORNL in 1997. Today, he is the leader of the lab’s Theoretical Physics Group, which encompasses both astrophysics and nuclear structure theory.
“If you want to understand how supernovae create elements, you better have the best supernova models you can get,” he said. “I came to ORNL to learn more about supernovae and use what I know about nucleosynthesis and radionuclei together.”
ORNL stood out because it was not just about theory and some of the best computational assets in the world, Hix said, but also had experimentalists at the Hollifield Radioactive Ion Bean Facility interested in nucleosynthesis who were open to collaboration.
“It was a nice synergy to have theory and experimentalists together and to be in a place that genuinely valued this kind of astrophysics,” he said. “The ecosystem of applied mathematicians, computational sciences and all the different kinds of expertise that you can access by walking down the hall is a real positive for the kind of work we’re doing here.”
An ecosystem of that size is needed when modelling something as massive and endlessly complex as a supernova explosion. There is a wealth of microphysics that go into every aspect of the models, Hix said, atomic physics data and nuclear reaction rates that must be measured in laboratories, data which is essential to calculating the models and interpreting the spectra collected by telescopes.
“Supernovae are fundamentally a multi-physics circus. It’s too much for any one person to keep all of it in their head,” he said. “It takes a village to make a supernovae model.”
When Hix first arrived at ORNL, the existing models only simulated the formation of the neutron star at the heart of a supernova, but failed to produce successful supernova explosions. Even up until about 10 years ago, he said, the best supernovae models in the world were still just two-dimensional slices.
“Imagine one wedge of an orange and compute just the wedge, then assume every other part of the orange is like that wedge,” he said.
Over the last two decades, computational astrophysicists have worked to create more sophisticated multi-dimensional models that would match with observations and allow them to explore the nucleosynthesis process. Today, modern computers can do full three-dimensional simulations with the same amount of physics on a larger and longer scale.
“The differences are significant, as you can imagine, because you end up with a completely disrupted star, and replicating one wedge of it all the way around doesn’t have all the degrees of freedom,” Hix said.
With bigger and faster computers, astrophysicists could afford to put in more complete physics and improve the dimensionality and resolution of the model. Traditionally models only simulate the first half second of the explosion, while newer versions can run for much longer and integrate better physics such as improved neutrino transport and three-dimensional hydrodynamics.
“That’s just the pieces of physics we already know we need, much less the other ones that we haven’t encountered yet,” Hix said.
Just as it takes a village to build a model, it takes an international community to make them better. Astrophysics is a global field, Hix said, with modelers working with other theorists, experimentalists, mathematicians, astronomers and computer scientists to obtain new data and solve each other’s seemingly intractable problems.
“You get the feeling that you’re contributing to human knowledge in the largest possible sense,” he said. “As much as research is about figuring out things nobody knows, half of the process is about telling the world because if you know it and nobody else does, you’re not done yet.”
Hix does his part to grow the research community through his work with students and early career researchers as a joint faculty member at the University of Tennessee. He enjoys teaching, he said, because of the curiosity and enthusiasm of the students new to the field and watching those students grow into sophisticated researchers capable of teaching him something new. It is those moments of mentorship—leading intern groups at the lab, helping grad students with their dissertation—that often give Hix the greatest sense of accomplishment.
“Every student I send out into the world means, in some sense, I’m doing more science,” he said. “It’s pushing back the darkness of ignorance, of not understanding our particular problem or other problems that these students choose to attack.”
UT-Battelle manages ORNL for the DOE Office of Science. The single largest supporter of basic research in the physical sciences in the United States, the Office of Science is working to address some of the most pressing challenges of our time. For more information, please visit www.energy.gov/science/.
