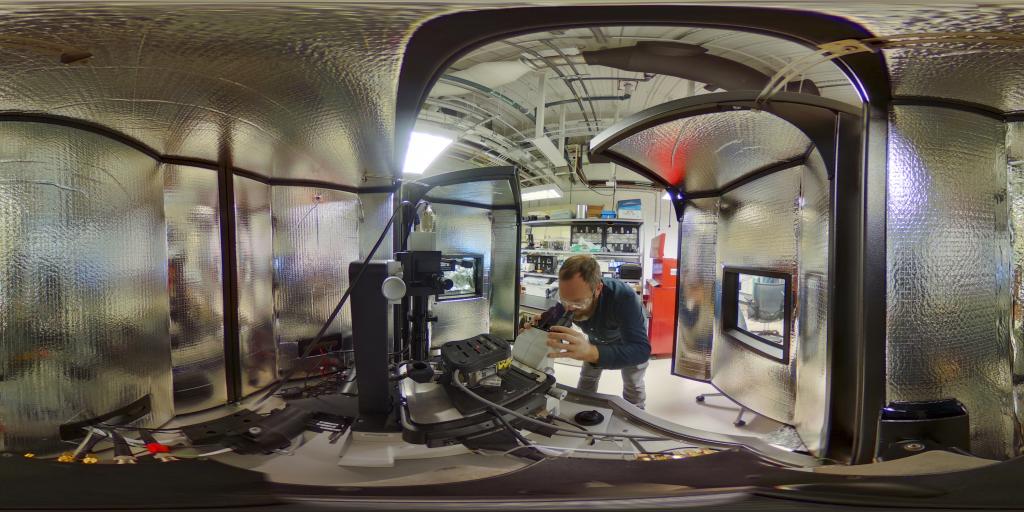
Liam Collins was drawn to study physics to understand “hidden things” and honed his expertise in microscopy so that he could bring them to light. Both interests converged at the Department of Energy’s Oak Ridge National Laboratory, where Collins focuses on materials—down to molecules and atoms—to answer fundamental questions about matter, motion and energy.
As a staff scientist at the Center for Nanophase Materials Sciences, a DOE Office of Science User Facility at ORNL, Collins supports the center’s user program by advancing microscopy techniques that push the limits of observation and enable researchers to study materials and their properties on a nanometer length scale.
CNMS users from all over the world work with Collins to gain nanoscale insights on materials that lead to new frontiers in energy, biology, medicine and beyond.
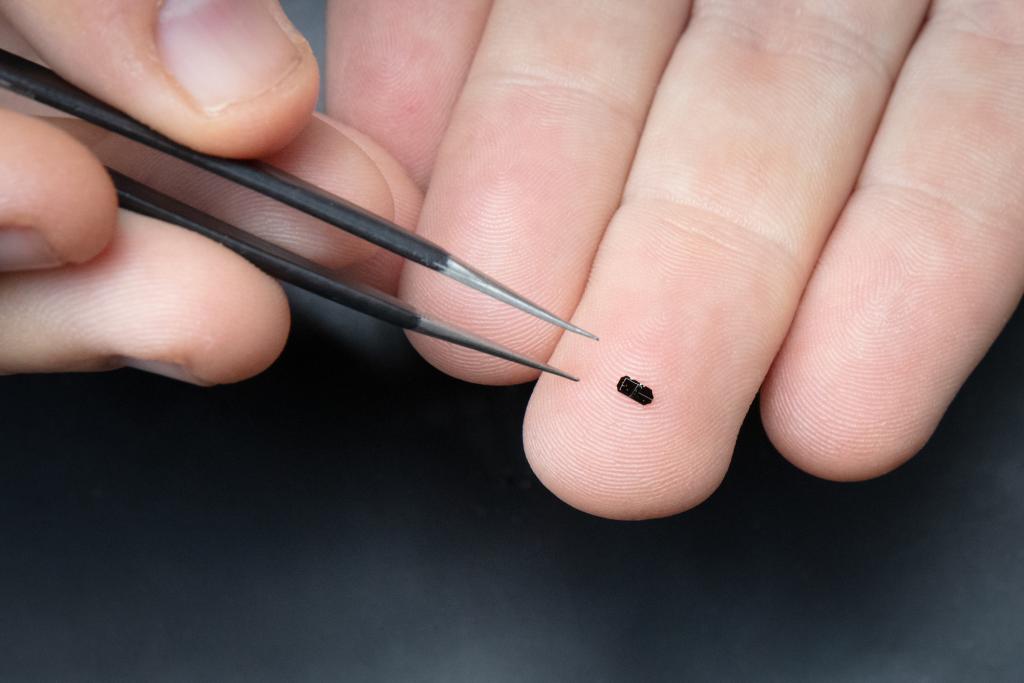
Material discoveries
Collins grew up in Ireland with a natural curiosity that sparked twinned desires for teaching and learning. The school he attended was small and its curriculum sparse, leaving the would-be physicist hungry for more.
“I’ve always been the kind of student—and still am—who wanted to know more, to ask the questions no one else had asked and to find out the answers no one else knew,” he said.
Collins’ passion for learning led him to pursue a teaching degree, with plans to expand science education in his hometown. He completed a bachelor’s degree at the University of Limerick and taught high school courses in a range of subjects, including physics, biology, agricultural science and math.
Teaching in turn whetted Collins’ appetite for research, and he went back to Limerick for a master’s in applied physics and then to University College Dublin for a doctorate in physics.
Collins’ UC Dublin research focused on advancing microscopy techniques to better understand biological systems. He set out to study biomolecular interactions thought to drive processes such as protein (mis)-folding, photosynthesis or even plaque formation in the brains of Alzheimer’s patients.
The challenge led him to Oak Ridge, where he connected with the lab as a CNMS user to develop novel atomic force microscopy techniques.
Atomic force microscopes (AFMs) are instruments that “see” by scanning the surfaces of materials with an atom-sharp probe to build up a picture of the nanoscale details—similar to the way a record player’s stylus feels the grooves in vinyl to play out music.
AFMs are powerful enough to feel the forces that bind molecules or move atoms—including mechanical, chemical, magnetic and electrostatic forces—and can map out the way a material responds to these unseen energies, capturing the relationship between structure and functionality.
Collins’ user projects resulted in several novel AFM techniques, jointly developed by UC Dublin and ORNL, that have made it possible to observe key energy processes taking place at nanometer and molecular levels in diverse materials.
Electrochemical force microscopy, for example, grew from an interest in seeing the surface charges of cell membranes to investigate their role in biological processes; it has since opened wider opportunities to study electrochemical and ionic processes at solid-liquid interfaces, including corrosion, sensing, energy storage and conversion.
“Once you are probing materials at the nanoscale and nearing the level of atoms, the kinds of questions you are asking will apply to many disciplines,” said Collins. “Whether users want to solve biological problems or improve the performance of batteries, they are on similar quests to understand and modify materials at the farthest reaches of science.”
Charting new territories
Collins’ user experience led him to extended collaborations with the CNMS, a subsequent postdoctoral appointment, and now a staff role in the Scanning Probe Microscopy group. Supported by the Office of Science, he furthers the CNMS aim to arrive first at new science frontiers and lay the groundwork for potential avenues of exploration.
“Our goal is to develop cutting-edge techniques that draw users who will carry the science forward,” said Collins.
Commercially available instruments like AFMs have made it possible for researchers to access nanoscale landscapes, but navigating the terrain is still an extraordinarily complex task. Materials can have multiple functionalities—electrical, chemical, mechanical—that occur simultaneously and evolve over time. Standard AFMs are powerful enough to observe the richness of the nanoworld, but the interconnected data they collect can be challenging to interpret.
“That’s why people come to the CNMS—because we are at the forefront of overcoming these obstacles to get unique insights with our instruments,” said Collins.
Techniques are really what drive the science, says Collins. “The instrument itself can give you a snapshot of materials on nano- and near-atomic scales, but you need to develop a range of techniques to capture and interpret the whole picture.”
Collins’ team at the CNMS pushes technology to capture previously inaccessible information, for example, “breaking the time barrier” to record fast dynamics that typically occur too quickly to be detected by off-the-shelf microscopes.
Another area of technique development addresses artifacts, or background noise—a known limitation of AFMs, which produce movements while scanning that can mask or interfere with sample measurements.
Collins recently demonstrated a quantitative method to overcome artifacts that contribute to false readings of electromechanical functionality in unexpected materials, even almonds (yes, the nut).
As a researcher, Collins gravitates toward the anomalies and complications encountered in materials science. His current areas of technique development focus on promising and complex materials in high demand among users, including mixed ionic electronic conductors and polymers. Both are trending for “hot” applications in electronics, robotics, memory and energy storage but are not well understood at the nanoscale.
“Confronting complexity is what leads us forward,” said Collins. “To advance materials for next-generation technologies, we will need to develop appropriate techniques to optimize their performance.”
Users, collaborators and colleagues, he says, all spark fresh ideas for adapting techniques to expand insights and make it possible to see or create something new. “That’s really why I’m here, to explore materials that challenge what we know and look for ways to deepen our understanding.”
UT-Battelle manages ORNL for the DOE Office of Science. The single largest supporter of basic research in the physical sciences in the United States, the Office of Science is working to address some of the most pressing challenges of our time. For more information, please visit https://energy.gov/science.
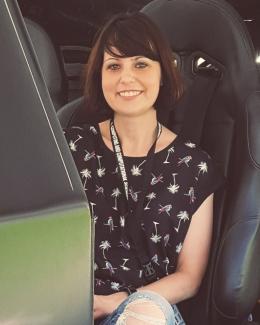