The research team poses in front of the airboat after a long day of research. Credit: ORNL, U.S. Dept. of Energy
As a biogeochemist at the Department of Energy’s Oak Ridge National Laboratory, Matthew Berens studies how carbon, nutrients and minerals move through water and soil. In this firsthand account, Berens describes recent fieldwork in Louisiana with colleagues. Their research focuses on advancing understanding of key processes in coastal ecosystems with the aim of improving models that predict the future of coastal environments in a warming climate.
Touchdown in Morgan City
The day began with a hot and humid sunrise along the Atchafalaya River in Morgan City, Louisiana. Team lead Beth Herndon and technician Geoff Schwaner woke up first and were immediately out the door to go for a run. Michael Jones, our other technical team member, went to find geocaches and pick up any missing supplies for the day. Because I was recovering from a running injury, I opted for a morning walk. I walked along the waterway and took note of the tall concrete levees that lined either side of the Atchafalaya, a poignant yet important reminder of the power of nature. As I passed through the city center, I came across a parking lot full of floats used in the Mardi Gras parade just days before we arrived. Everything from the local wildlife to the countless restaurants claiming to have the best crawfish boils in Louisiana was new to me.
We came to the Louisiana Gulf Coast to perform research in support of Beth’s DOE Early Career Research award, titled, “Biogeochemical Controls on Phosphorus Cycling in Urban-Influenced Coastal Ecosystems.” The goal of the project is to evaluate how climate change and human activities shape the biogeochemistry of coastal wetlands and influence the amount of nutrients flowing into the ocean. This information is vital for understanding how the functioning of healthy ecosystems will be impacted by environmental change in parts of the country that are experiencing the compounding effects of sea level rise and coastal degradation. The knowledge can be used to improve predictive modeling capabilities and potentially inform future coastal restoration projects.
Airboats, alligators and aquatic ecosystems
Our 45-minute commute by boat was the perfect opportunity to take in the sights, sounds and smells of the Louisiana Gulf Coast — the lush hardwood swamps, the numerous species of migratory birds and the steady hum of barge traffic. Our boat ride took us first along the Gulf Intracoastal Waterway, a 1,050-mile-long artificial shipping canal that spans the Gulf Coast from Texas to Florida. Completed in 1949, the Intracoastal Waterway is the nation’s third busiest waterway; the portion through Louisiana alone supports five of the top 15 busiest shipping ports in the United States.
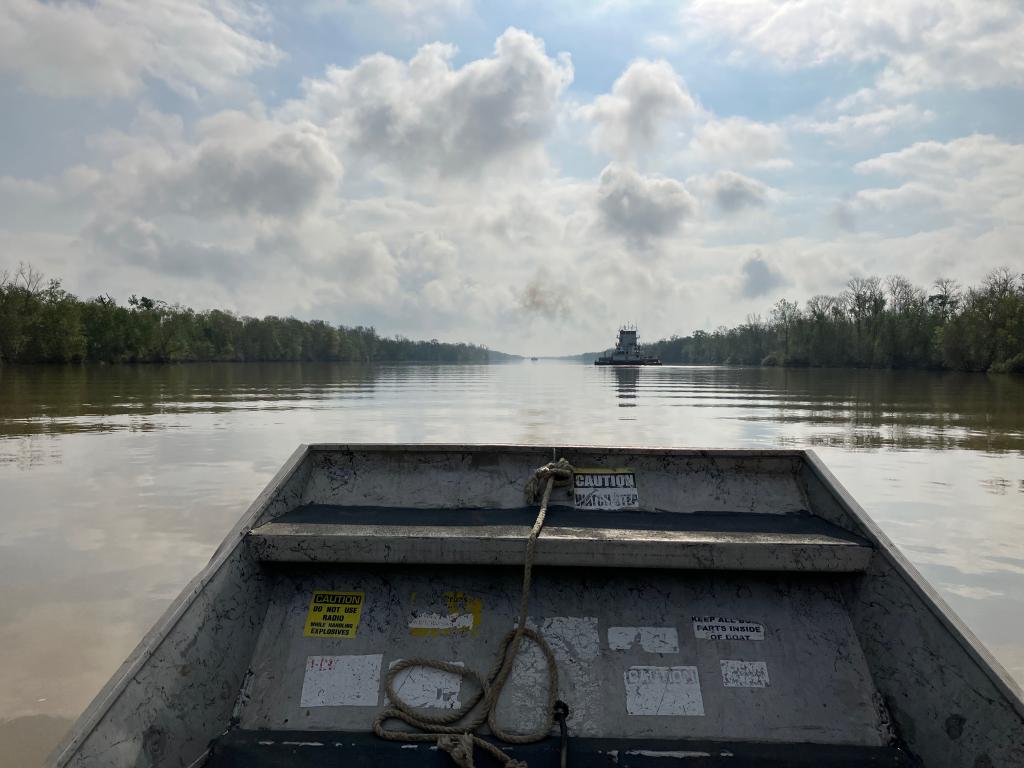
It was fun, yet daunting, to be directly alongside barges over 200 feet long. Our boat then turned south down the Wax Lake Outlet into an area teeming with wildlife. We frequently spotted double-crested cormorants, roseate spoonbills, bald eagles and American alligators.
When we arrived at our study site, we put on our waders and got to work unloading our gear. The site is located in the Wax Lake Delta, a nutrient-rich freshwater delta that began forming in the 1940s after a canal was dug to direct flow from the Atchafalaya away from flood-prone areas of the Louisiana coast and into the Gulf of Mexico. The sediments carried in the river began depositing where the land meets the ocean, forming islands that are part of what is now the Wax Lake Delta.
At a time when much of the Gulf Coast is deteriorating from the widespread impacts of sea level rise, the Wax Lake Delta is one of the only actively growing areas of the Louisiana coast. This gives our team a unique perspective to study ecosystems that could potentially develop in response to current and future restoration projects.
When viewed on a map, our field site looks like a well-defined island in the delta. In reality, it is a complex array of tree-lined berms, tidal wetlands and open water that can transform dramatically in a matter of hours due to tidal fluctuations and changes in weather. This meant that our working locations were often partially or completely flooded. Thankfully, our airboat could drive over water, mud, vegetation and even dry land to transport our gear safely to the more challenging spots.
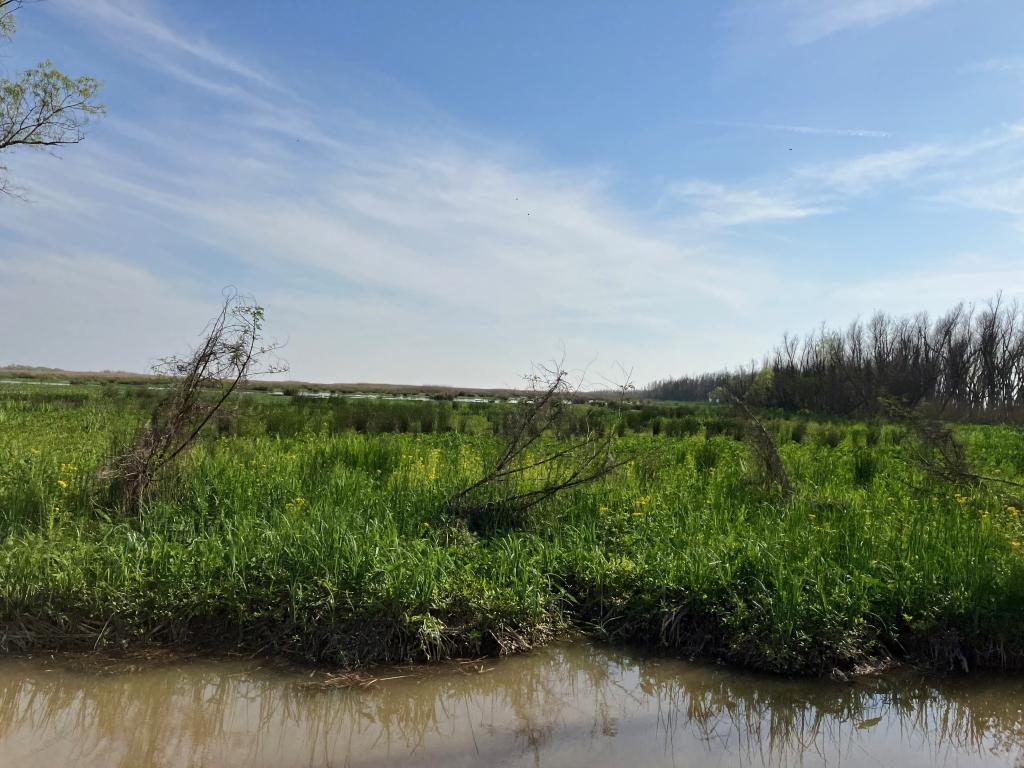
As a Minnesotan, I was unfamiliar with the concept of an airboat. I am more accustomed to small motorboats, canoes or even the occasional snow machine. Riding on the airboat was one of the favorite activities shared by our team.
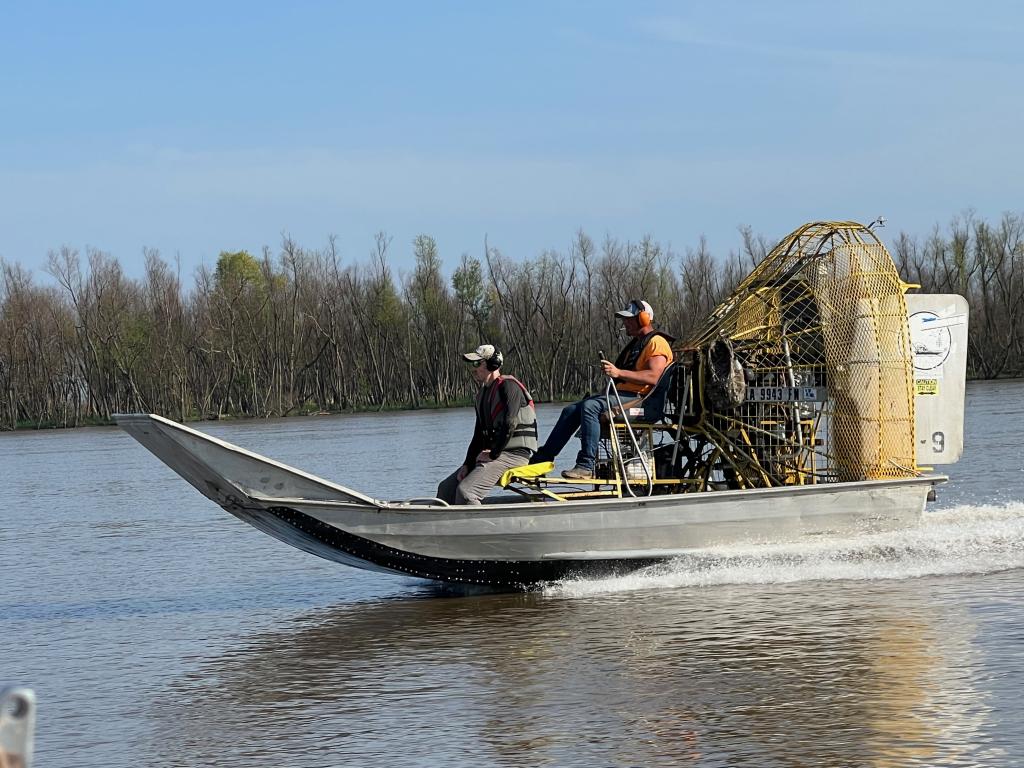
Establishing long-term, nutria-resistant monitoring
Our first task was setting up monitoring equipment that will take continuous measurements of the temperature, pH, salinity and reduction-oxidation potential of the groundwater over the next several years. Because the sensors need to be installed in the soil, we used plastic pipe to make 1-meter-deep wells that place the sensors in direct contact with the groundwater while keeping them separate from the surrounding soil.
One peculiar challenge of installing the sensors was protecting the hundreds of feet of cable from the gnawing teeth of nutria. If, like me, you are unfamiliar with nutria, think of a 20-pound cross between a beaver and a rat, with giant orange teeth and a long, hairless tail. To deter these creatures, we put all cables inside thick plastic conduit. This was a surprisingly difficult and frustrating process, but Michael turned out to be extremely skilled and efficient at this task.
While Beth and I finished installing sensors, Geoff and Michael began constructing elevated platforms to hold the data storage devices and solar panels to power the equipment. The platforms were built by attaching wooden boards to long metal poles inserted into the ground, similar to putting in a dock. It was not easy to build a structure on the soft, mucky ground, but Geoff and Michael did an excellent job creating a sturdy foundation. Once the platforms were installed, we connected our equipment and could finally make sure everything worked — and then breathe a sigh of relief. The frogs nearby seemed to narrate our progress as we worked each day.
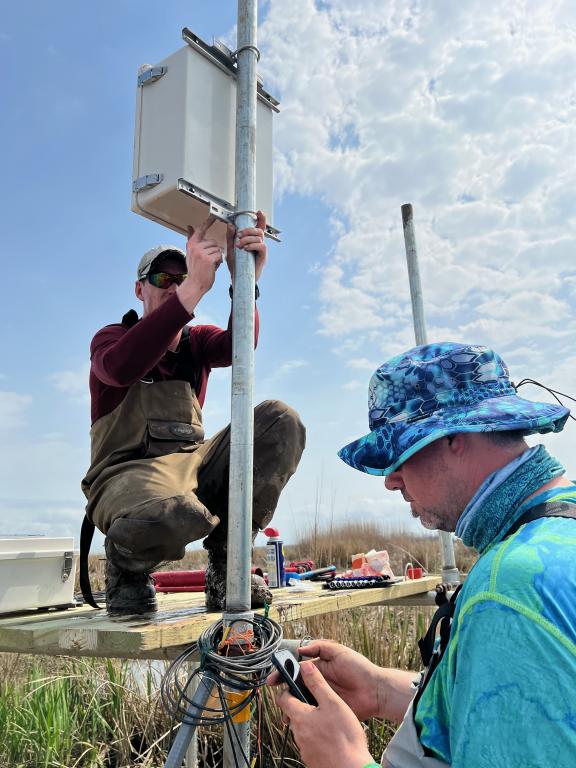
“Sipping” the soil water
Our final main task for the week was collecting samples of porewater, the water present in the open spaces beneath the soil surface. Throughout a complex network of solid soil particles and open flow paths, this porewater interacts with different soil minerals and variable oxygen levels to create unique patterns of belowground water chemistry. These belowground patterns play perhaps the biggest role in influencing the overall biogeochemistry of the coastal environment. To capture these trends, we used “sippers” to extract water from multiple depths below the soil surface.
Sippers are long plastic tubes with a small opening at one end and a syringe connected to the other. By inserting the sippers into the soil, we were able to measure how the water chemistry changes from the surface down to 50 centimeters below ground, about 20 inches. These sippers were all homemade. In the weeks leading up to the trip, Geoff spent countless hours constructing more than 30 sippers, each of which was carefully crafted to ensure that its length was accurate and the openings were big enough to allow water through without it filling up with sediment and debris.
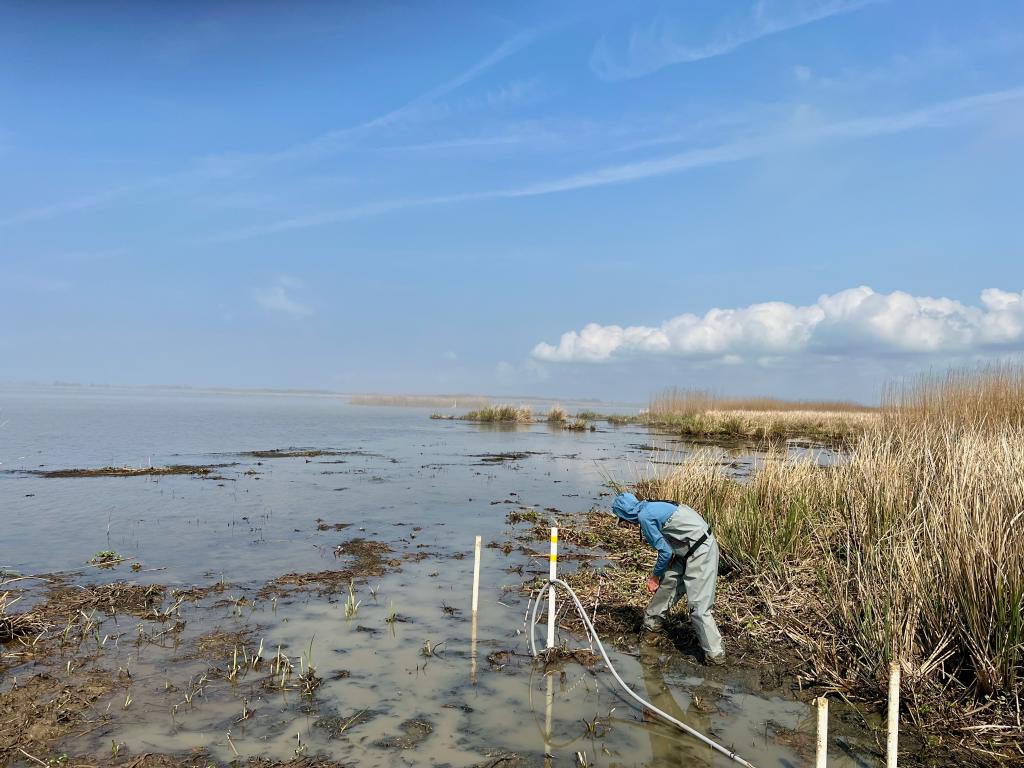
Future research
After each day of work, we packed up our equipment and began the 45-minute boat ride back to the car. Tomorrow would be another hot and humid day, bringing with it the excitement of new adventures in science! This trip was only our first of many planned visits to the Wax Lake Delta in the coming years.
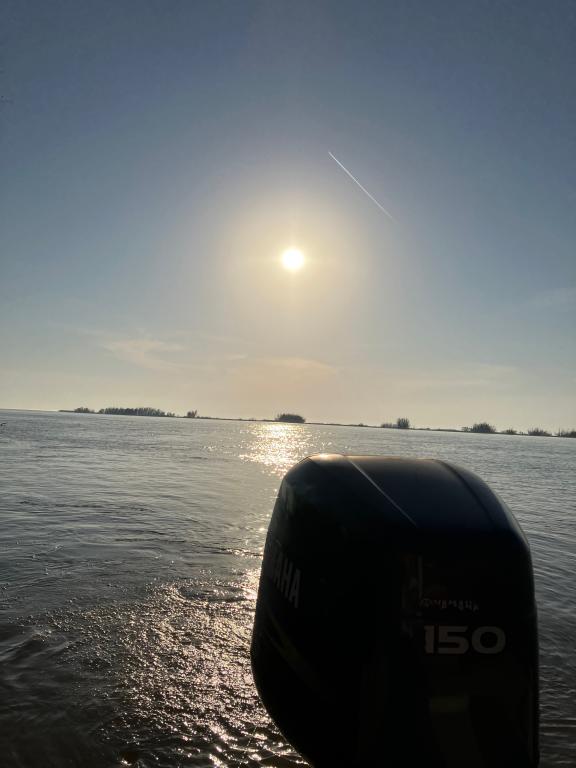
In collaboration with our partners at Louisiana State University, we plan to visit the site several times throughout the year to check on our monitoring equipment, download data and collect more porewater and sediment samples. I am fortunate that my postdoctoral appointment began around the same time as the project, meaning that I will get to see it through almost from beginning to end. I have so far learned an incredible amount from Beth and the rest of the team, and I am grateful and excited to continue working on this project as a member of ORNL’s Environmental Sciences Division.
This research is supported by the Biological and Environmental Research program in DOE’s Office of Science.
UT-Battelle manages ORNL for the Department of Energy’s Office of Science, the single largest supporter of basic research in the physical sciences in the United States. The Office of Science is working to address some of the most pressing challenges of our time. For more information, please visit energy.gov/science. — Matthew Berens