FRIB: Bringing Cosmic Elements to Earth
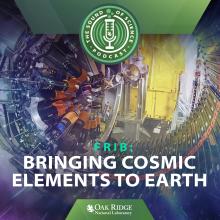
When the universe was formed billions and billions of years ago, the building blocks of life were forged with it. Hydrogen, carbon, iron, nitrogen, calcium, oxygen are just a few of the elements born from the cosmos that make up life on Earth. There are currently 118 known elements and we use the periodic table to organize and understand them. But straightforward as the periodic table seems, it contains a lot of mysteries. Scientists have catalogued more than 3,000 known isotopes and speculate there are thousands more yet to be discovered. The Facility for Rare Isotope Beams, also known as FRIB, has come online at Michigan State University to help researchers in their quest to create new isotopes and study their exotic behavior. FRIB has been years in the making. In fact, several of the instruments and detectors used originated from a historic facility at Oak Ridge National Laboratory. In this episode, you'll hear from several scientists who have been along for the journey about the history and future of this new facility – and what it means for science and society.
Transcript
[THEME MUSIC]
ALLMOND: It's basically like having a new set of eyes, the view of the world.
NAZAREWICZ: Patience is very important here. We never lost hope. There were many detours. Lots of heart attacks on the way.
CHIPPS: We’re all made out of these atoms. And we want to understand where they came from, because it tells us something about where we came from.
[MUSIC TRANSITION]
JENNY: Hello everyone and welcome to “The Sound of Science,” the podcast highlighting the voices behind the breakthroughs at Oak Ridge National Laboratory.
MORGAN/JENNY: We’re your hosts, Morgan McCorkle and Jenny Woodbery.
[MUSIC TRANSITION]
JENNY: When the universe was formed billions and billions of years ago, the building blocks of life were forged with it.
MORGAN: Hydrogen, carbon, iron, nitrogen, calcium, oxygen are just a few of the elements born from the cosmos that make up life on Earth – plants, animals and even humans.
JENNY: Renowned astrophysicist Carl Sagan once said “we are all star stuff,” because the elements in our own bodies can be traced back to the founding of the universe.
MORGAN: We use the periodic table to organize and understand all these elements, as they are the basis for everything in our world.
JENNY: There are currently 118 elements on the periodic table. Ninety-two of these are naturally occurring – meaning they originated with the formation of the universe. The other 26 are manmade -- produced in laboratory experiments involving nuclear reactions.
MORGAN: As straightforward as the periodic table seems, it contains a lot of mysteries. Each element is determined by the number of protons, or positively charged particles, in its nucleus. But the number of neutrons – neutral particles also located in the nucleus – can vary, forming what are called isotopes of the same element.
JENNY: Scientists have catalogued more than 3,000 known isotopes and speculate there are thousands more yet to be discovered.
MORGAN: The Facility for Rare Isotope Beams, also known as FRIB, has come online at Michigan State University to help researchers in their quest to create new isotopes and study their exotic behavior.
JENNY: FRIB has been years in the making. In fact, several of the instruments and detectors used originated from a historic facility at Oak Ridge National Laboratory.
MORGAN: We talked to several scientists who have been along for the journey about the history and future of this new facility – and what it means for science and society.
[MUSIC TRANSITION]
SAMUEL STANLEY: Three, two, one. Cut! [Applause]
JENNY: Energy Secretary Jennifer Granholm and MSU President Samuel Stanley officially opened FRIB on May 2, 2022, making it the newest U.S. Department of Energy Office of Science user facility.
MORGAN: DOE user facilities give scientists from around the globe the opportunity to use state-of-the-art tools like supercomputers, research reactors, light sources and more to advance scientific discovery.
JENNY: FRIB joins this line up as a powerful particle accelerator capable of creating the rare isotopes we mentioned earlier.
WITEK NAZAREWICZ: FRIB hosts now the most powerful heavy ion accelerator in the world and thanks to the beams from FRIB, over 1,000 newer isotopes will be created. Those isotopes do not exist in nature, because they are radioactive, they decay, but those isotopes are very important for our understanding of what is happening on Earth, what is happening in the cosmos, and it's also important for various societal applications.
MORGAN: That’s Witek Nazarewicz. He is FRIB’s chief scientist and is a John Hannah Distinguished Professor of physics at MSU.
JENNY: Witek and his colleagues in the nuclear physics community have waited a long time to see FRIB become a reality.
NAZAREWICZ: If you think about it, the first ideas were in 1989. Thirty-three years. Lots of convincing had to be done to convince our colleagues, scientists and nuclear physicists, right, because the amount of funds is always very limited. So, patience is very important here. Okay, we never lost hope. There were many detours, many roller coasters on this path to FRIB. You know, lots of lots of heart attacks on the way.
MORGAN: While there were challenges along the way, FRIB is finally online and is enabling scientists to explore the field of nuclear physics like never before.
[MUSIC TRANSITION]
JENNY: So just how do you create cosmic isotopes on Earth?
MORGAN: At FRIB, the process starts with a heavy-ion beam traveling at more than half the speed of light through a 1,600-foot, paperclip-shaped linear accelerator. When the beam reaches the end of the accelerator, it strikes a rotating graphite target – setting off a nuclear reaction.
MITCH ALLMOND: That basically shatters the nucleus and creates a cocktail -- many different residual nuclei, many of which are unstable and very exotic, normally only seen in the universe and astrophysical phenomenon like supernova and neutron star mergers. This cocktail of unstable nuclei can then be separated in this long device that basically filters out the isotopes of interest and can then deliver it to an experimental end station that has a suite of detectors. And so FRIB will provide us unprecedented access to these exotic nuclei.
JENNY: That’s Mitch Allmond, he’s a physicist at ORNL who studies low-energy nuclear structure.
MORGAN: The instruments at FRIB will help scientists make discoveries about the properties of rare isotopes, nuclear astrophysics, fundamental interactions and applications for our everyday lives.
JENNY: Mitch is the project manager for one of those instruments known as the FRIB Decay Station initiator, or FDSi.
MORGAN: FDSi is basically a collection of detectors that is extremely sensitive to rare isotope decay signatures.
ALLMOND: We wanted to maximize the scientific output opportunities. To do so, we decided that what we needed was this FRIB Decay Station Initiator. It's a mechanical infrastructure that's designed in a way to provide this integration of all the community detectors into a single platform ecosystem.
JENNY: FDSi has a unique design that allows scientists to quickly mix and couple detectors to capture different types of nuclear data.
ALLMOND: We also wanted to be able to reconfigure these resources quickly, without losing beam time or spending too much of our time with having to basically reconstruct and deconstruct, and all that so it has a reconfigurable infrastructure to where we can do multiple different measurements that might require a different ensemble of detector types. There's many different ways that we can combine detectors to answer different questions efficiently.
MORGAN: While FDSi is new to FRIB, it and several other instruments at the facility grew out of pioneering work at ORNL to create beams of radioactive ions. This research was done at the Holifield Radioactive Ion Beam Facility.
JENNY: The former DOE user facility once gave scientists access to high-quality beams of 200 rare isotopes.
MORGAN: Robert Grzywacz, a University of Tennessee physics professor who holds a joint appointment at ORNL, spent many years at Holifield and was part of the research that laid the foundation for FDSi.
ROBERT GRZYWACZ: This is a collaborative endeavor, which includes many detectors from multiple labs to put in a sort of unified infrastructure. It does have a Holifield origin, because if you look into the instrumentation, there's plenty of instruments which were developed at the kind of end of Holifield. And so can recognize them to be used in in the last experiments at this facility.
JENNY: One of Robert’s colleagues at the facility was someone you’ve already met in this episode.
MORGAN: Before joining MSU, Witek Nazarewicz was a corporate fellow at ORNL and led the Holifield facility.
JENNY: Robert and Witek’s research at Holifield helped advance detector technology that can now be found in instruments like FDSi.
GRZYWACZ: So, one of the things which we, I believe pioneered at Holifield was the use of the so-called digital electronics, which dates back to, essentially the year 2000, when we first started to use it, in the very in this proton emission discovery experiment. And the so we kind of dived into this technology, it was very, very new. And to some degree rather painful to use. But I think over about a decade, we made it really to be mature method.
MORGAN: While Holifield was decommissioned in 2012, the cylindrical tower that housed the facility’s vertical linear accelerator has become an iconic piece of the lab’s landscape, and the technology pioneered there lives on at FRIB.
JENNY: Robert developed a new instrument for FRIB that is used in tandem with FDSi called the Versatile Array of Neutron Detectors at Low Energy, or VANDLE. It provides energy information about neutrons.
MORGAN: FDSi-VANDLE were part of the first experiment run at FRIB, which was led by Lawrence Berkeley National Laboratory with collaborators from ORNL and the University of Tennessee, Knoxville.
JENNY: The team used a variety of sensitive detectors to make first measurements of survival times for five exotic isotopes.
ALLMOND: This very first paper is more or less the question of how long do they live. And so, in the first paper, we published the half-lives, which the half-life is essentially the time it takes for an ensemble to decay by half. So, if I had 100 of them, you know, how long would it take before I only had 50 of them? That’s a simple definition of a half life. In this paper, we reported five new half-lives for the first time that weren't known before. Of course, the half-lives in themselves are interesting, because it does challenge the state-of-the-art nuclear theory, right, because state-of-the-art nuclear theory tries to predict these half-lives. It's clear that there's still deficiencies in the theory, the theory isn't able to explain what we experimentally observed. So, there's still room to improve the theory and improve the physics input to how these systems are formed and how they fall apart or decay into something else.
MORGAN: This initial publication is only the beginning for Mitch and his colleagues. The team already has a second paper in the works that further analyzes the results from FDSi-VANDLE.
ALLMOND: These five new half-lives, and then of course, confirming previous half-lives, was kind of like the first step, the simplest first step that we could take to process and report the data, but then we’ll have extra stages, there's already a second paper that's been developed. And then there's many more papers that will come and report things on --what happened to the neutrons? How did they decay? You know, what about all the gamma rays? What are they doing? What does it tell us about the nucleus? Does it tell us something about the shape of the nucleus is deformed or spherical, and various other kinds of characteristics of the nucleus. So, these subsequent papers will touch on some of these other topics and get a little bit more detailed in the spectroscopy that we're doing. And a little bit more detailed on the theory and, of course, continue to challenge theory and update theory to kind of get the physics correct. Based on the survey of data we have taken, it’s clear that the combination of FRIB and the FDSi, it's basically like having a new set of eyes, the view of the world, you know, it's, there's a lot of stuff, and it's gonna take a long time to deconvolute it all and to understand it all, but it's clear that this is a significant milestone and there'll be a lot more to come.
[MUSIC TRANSITION]
JENNY: In addition to basic laws of physics, scientists are using FRIB to gain new insight into stellar explosions and the origin of life.
MORGAN: One instrument at the facility is uniquely suited to help answer these questions, the Jet Experiments in Nuclear Structure and Astrophysics, also known as JENSA.
JENNY: The design of JENSA was led by Kelly Chipps, a nuclear astrophysicist at ORNL.
KELLY CHIPPS: Usually when you're looking at these reactions that are taking place in stars, a star is made up mostly of hydrogen and helium. Hydrogen and helium are gases here on Earth. And so, it's not easy to kind of collect them all into one place and use them for these nuclear reactions, because they're a gas and the gas wants to expand everywhere.
MORGAN: She and her team took this challenge into account when developing the target for the instrument.
CHIPPS: JENSA is very cool, because it uses a high pressure of that gas into a very specific nozzle. And this little nozzle basically acts like a fire hose, it turns that gas into this really just perfect stream, very dense. But it's very localized, it stays in that one spot, just like the water coming out of a hose. So instead of spraying everywhere, like a sprinkler, it's coming right out in that stream, just like a hose. And that means that it creates a perfect target for you to hit it with your radioactive ion beam and do a nuclear reaction. So, without there being anything there to have to contain the gas and hold it in, you know, like a balloon or like windows of some type. It's really just the gas. And it's almost as dense as a solid. It’s the only one in the world. That is that dense.
JENNY: While JENSA hasn't been involved in the initial experiments at FRIB, Kelly and her team were able to test it out using one of the ion sources at the facility.
CHIPPS: Right before FRIB started up, we were using an ion source that provides long-lived radioisotopes. One of the nice things about this facility is that you can have a beam from the accelerator that is going to a particular end station, a particular experiment. And at the same time, you can have a beam from an ion source that's going to a different experiment. Those things can run in parallel and that's a real boon for us, because it means that you can fit more people into you know, a schedule than you would be able to otherwise, that gives more people the opportunity to do science.
MORGAN: The results from the ion source experiment yielded some interesting results and are just a preview of what is to come by using JENSA on the full accelerator.
CHIPPS: So that was what we were doing with JENSA, we were running a beam of aluminum-26, which has a half-life of almost a million years, it's 700, and some 100,000 years. And the interesting thing about aluminum-26 Is that you can see it in the galaxy, there are space-based telescopes, like Integral and COMPTEL that can actually map aluminum-26 from its decay, it decays via a gamma ray, a very characteristic gamma ray. And they can see that in the galaxy, and they can actually pinpoint where it's coming from. So, we know that it's there. 700,000 years is a long time for us. But it's a very short timeframe for the life of a star. So, we know that it's being actively produced in the stars, because if it was produced only when the star was born, that it would all be gone by now. So, understanding how the aluminum-26 is being produced and destroyed in these stellar environments is going to tell us something about that star; it’s going to tell us how that star is burning, how fast it's burning, how hot it's burning, what sorts of nuclei it's producing. So that was the last thing we did, which was really cool.
JENNY: FRIB will be accepting proposals for both the accelerator and the ion sources, which will allow more users to have access to the facility.
MORGAN: And speaking of users, Kelly not only leads the JENSA experiment, but is also the chair of the FRIB User Organization.
CHIPPS: The FRIB users organization has existed since roughly 20 years now. The community basically came together at that point in the early 2000s, and came to the conclusion that what was needed in the community was a new facility that was going to allow us to reach toward, really the extremes of the nuclear chart. So all sorts of nuclei that don't exist anywhere on Earth, things that are at the limit of what is even bound, which means that past that point, all of the nucleons just start falling apart, they don't, they just can't exist together anymore. This was something that was seen as a very high priority within the community. And so the community started getting together, having town halls and white papers and meetings, formed the users organization as kind of a, you know, a group to make this a more coherent project. And then after the project itself was awarded by DOE, this was our way of making sure that the community was engaged with the project and helping to define the science goals and the kinds of equipment that we were going to need to be able to achieve those goals and the kinds of expertise that was going to be needed both on the experimental side and on the theoretical side.
JENNY: The user community’s executive board holds an election each year to choose its chair and this year, that person was Kelly.
CHIPPS: Basically, In this position, I act as a liaison between the user community and the facility management. So, if users are interested in seeing something happen, from a scientific standpoint, you know, they want to see the development of a certain piece of equipment or a certain type of radioactive beam. If they have questions or concerns, that kind of comes to me, and then I come to the FRIB management, and I say, on behalf of the users, you know, here are these questions, or here are these priorities. So, it's definitely not something that's for the faint of heart. It's a lot of users that the users group at the moment is just shy of 1,600. So, there's, there's a lot of community engagement. And it's really nice to see there's a lot of community engagement, but it also means that there's a lot for me to do.
[MUSIC TRANSITION]
MORGAN: It’s been a little over six months since the facility opened, and the excitement is still palpable.
JENNY: Kelly gave us some insight into what it was like to be at FRIB during the initial experiments.
CHIPPS: We’re looking to see isotopes that nobody has ever measured before. And I think that there was a lot of excitement around that. And you could tell because everyone is clustered, you know, in the control room, you have only a couple, you know, monitors that are that are showing the data as it's coming in. But there's, you know, 30 or 40, people trying to just, you know, pack around those couple of monitors and see things as they're happening. So it was, it was really exciting. And it's I've never seen that many people, you know, in that room at one time. Like, just to see that level of excitement. And everybody being in, waiting with bated breath to see these events come in kind of, you know, one at a time. And some of these things, right, like I said, they've not been seen before. So, we don't know what the half-life is, we don't know how long it lasts, we don't know how many of them we're going to see. And so you're waiting, and then you see, you know, one point show up on the screen. And, and then five minutes later, there's another point on the screen, and everybody's just, oh my gosh, like there's another one. And I've been on experiments where people were so excited that they started naming the individual events and like this one is Jim and that one is Sherry. And this is Janis. It's that level of just this emotional investment in the science, which is really nice to see. Because it's not just a job it's something that we care very deeply about and we love doing.
MORGAN: The facility may be brand new, but upgrades to the instruments and power level are already in the works. That’s something Mitch Allmond is particularly excited about.
ALLMOND: And so there's always a little bit of a foot in the future, in the unknown, and a foot and in the past, and what did you learn from before, and how can you make the current and future runs better. And of course, we're still developing phase two of the FDSi, that'll be implemented in this next summer. There’s a lot of things happening. We’re trying to use phase 1 of FDSi, we’re trying to design and build phase 2 at the site to be implemented in the summer, and we're trying to learn the various nuances of the new facility. In terms of what am I looking forward to, I'm looking forward to the FDSi to be finished and fully implemented at the point where we can operate it more, you know, more routine fashion. And, of course, looking forward to the facility being complete. So, the facility is having to stage itself with respect to beam intensity, mostly for just kind of safety protocol reasons. So, you know, when we began, the machine started off at 1 kilowatt of power. And so we'll be running again in the April timeframe of 2023. And I believe we'll be up to 6 kilowatts of power, and maybe by next year, it will be at 10 kilowatts of power. But ultimately, we have to get up to 400 kilowatts of power. A lot of what we’re really interested in needs the 400 kilowatts of power. We’re doing the best we can with the limited power we have. And there’s a few niche cases here and there that we can do that provides new physics and new results and discoveries.
JENNY: And for Witek Nazarewicz, he’s waited 30 years to see this facility come to fruition, and now that it’s here, he knows he may have to wait a little longer until they see groundbreaking results, but it will be worth it.
NAZAREWICZ: The first part of the FRIB program has to be doable. You have to run experiments which will be successful. You have to run experiments which will result in first class papers. Those are not the most challenging experiments which are going to be run at FRIB. So, we would like to start slow, so there will be decay experiments, there will be experiments related to nuclear astrophysics, right. So, with time, I hope that within a year, we'll be reaching to the extremes, that there will be experiments dealing with extremely exotic nuclei -- when we say extremely exotic nuclei we mean those which have this most abnormal ratio of neutrons to protons. Nucleus is made of neutrons and protons and normal nuclei which surround us – 286 stable nuclei around us -- they have very specific ratios of neutrons to protons, but those exotic ones which will be produced at FRIB will have crazy ratios of neutrons to protons. So, the calcium, stable calcium isotopes, which exist in nature, like calcium 40 or calcium 48, 20 protons, 20 neutrons, 20 protons, 28 neutrons, but FRIB hopefully will take us all the way to calcium 70, which has 20 protons and 50 neutrons -- a dramatic excess of neutrons of over protons, right. So, and for physicists such as myself, this is an extremely exciting proposition, because it will take us to atomic nuclei, which have this abnormal density of neutral matter, you know, which you can see, of course, in different conditions in neutron stars. So, neutron-rich nuclei can tell us a lot about neutron stars. So, I get easily excited.
MORGAN: And although understanding neutron stars may seem far removed from our everyday lives, Kelly Chipps reminded us that the research at FRIB is helping explain who we are – at the most fundamental level.
CHIPPS: And so, it becomes a part of a whole suite of tools that we have to really understand the basic fundamental nature of the nucleus. And from that point of view, it's really exciting because that's what we're all made out of. We’re all made out of these atoms. And we want to understand where they came from, because it tells us something about where we came from.
[MUSIC TRANSITION]
JENNY: Thank you for listening to this episode of The Sound of Science.
MORGAN: We hope you enjoyed this episode and leave us a review wherever you get your podcasts.
JENNY: If you want updates on the amazing science that will be coming out of FRIB, follow @FRIBLab on Twitter, or visit frib.msu.edu.
MORGAN: Until next time!