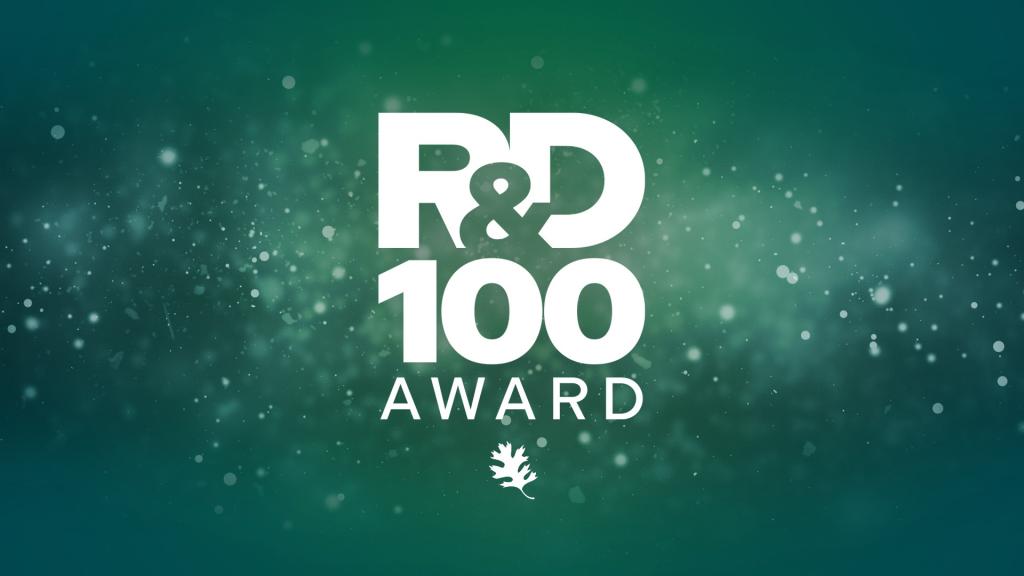
In an impressive showcase of cutting-edge innovation and scientific prowess, Oak Ridge National Laboratory has been recognized as a beacon of technological excellence, receiving 14 R&D 100 Awards, announced this week by R&D World magazine.
This year’s winners represent not only a pinnacle of research but also embody ORNL’s relentless pursuit of innovation that drives progress across industries.
“ORNL researchers push the edge of innovation,” said ORNL Director Stephen Streiffer. “The R&D 100 Awards showcase the far-reaching and impactful work they do to address national challenges through science and technology.”
R&D World magazine announced the winners from a selection of 141 finalists representing 16 countries and regions. ORNL either led or played a role in 24 of the projects named as finalists. The winners will be recognized at the organization’s award ceremony November 21, 2024, in Palm Desert (near Palm Springs), California.
Often referred to as “The Oscars of Innovation” and the “Nobel Prize of Engineering,” the R&D 100 Awards has for the last 60-plus years honored science and technology research and development leading to new commercial products, technologies and materials that are available for sale or license.
ORNL’s R&D 100 Award winning technologies and their developers are:
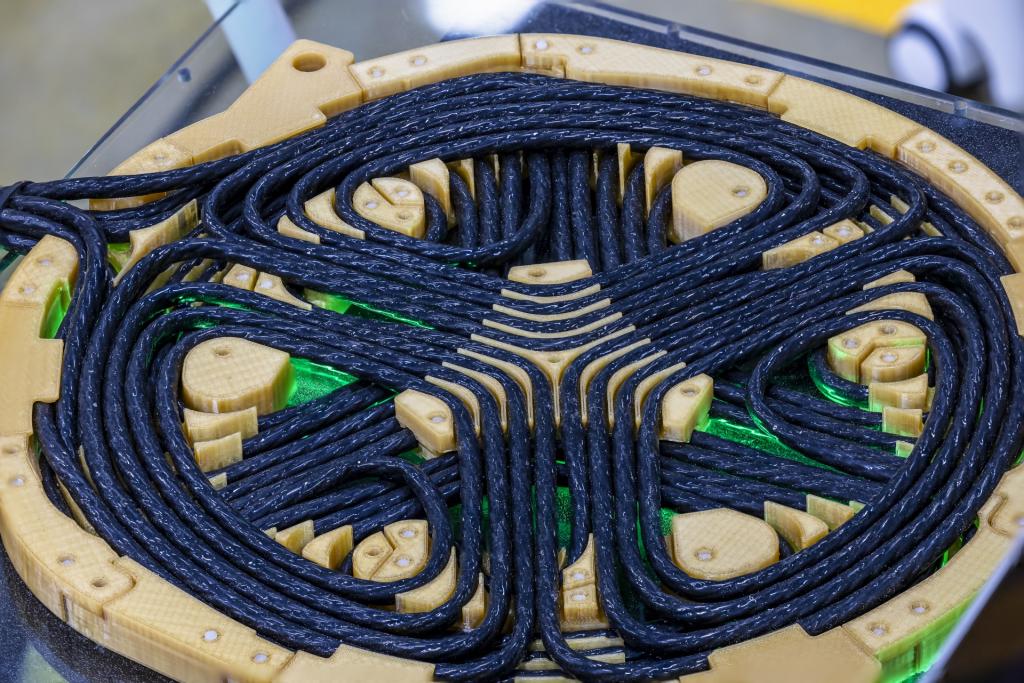
Polyphase Electromagnetic Couplers for Extreme Fast Wireless Charging of Electric Vehicles
Polyphase electromagnetic wireless charging includes the most compact and lightweight coils possible to achieve the highest-power transfer levels to electric vehicles, or EVs. This process is similar to the wireless charging of small consumer devices, but the unique geometry and design of the polyphase coils enable the transfer of extremely high-power levels using rotating magnetic fields generated by the coil phase windings to boost the power.
ORNL’s research team demonstrated a 100-kilowatt wireless power transfer to a Hyundai Kona EV and a 270-kW wireless power transfer to a Porsche Taycan EV – both world records for light-duty passenger vehicles. This technology also achieved power densities 8 to 10 times higher than existing systems. Power density is important in wireless charging because it indicates how much power the system can handle relative to its size. The goal is to achieve higher densities from smaller, more compact systems to improve overall efficiency and performance. These charging durations and power densities bring electric vehicles much closer to the conventional gas station refueling experience.
The research was funded by DOE’s Vehicle Technologies Office in the Office of Energy Efficiency and Renewable Energy.
The technology was developed by ORNL scientists Omer Onar, Mostak Mohammad, Emrullah Aydin, Gui-Jia Su, Jonathan Wilkins, Larry Seiber, Cliff White, Clayton Hickey, Veda Galigekere, Burak Ozpineci and Rich Davies; Hendrik Mainka, Ruediger Kusch and Andrew Foote of Volkswagen Group; Kenneth Gould of Porsche Cars North America; and Rakan Chabaan of Hyundai-Kia America.
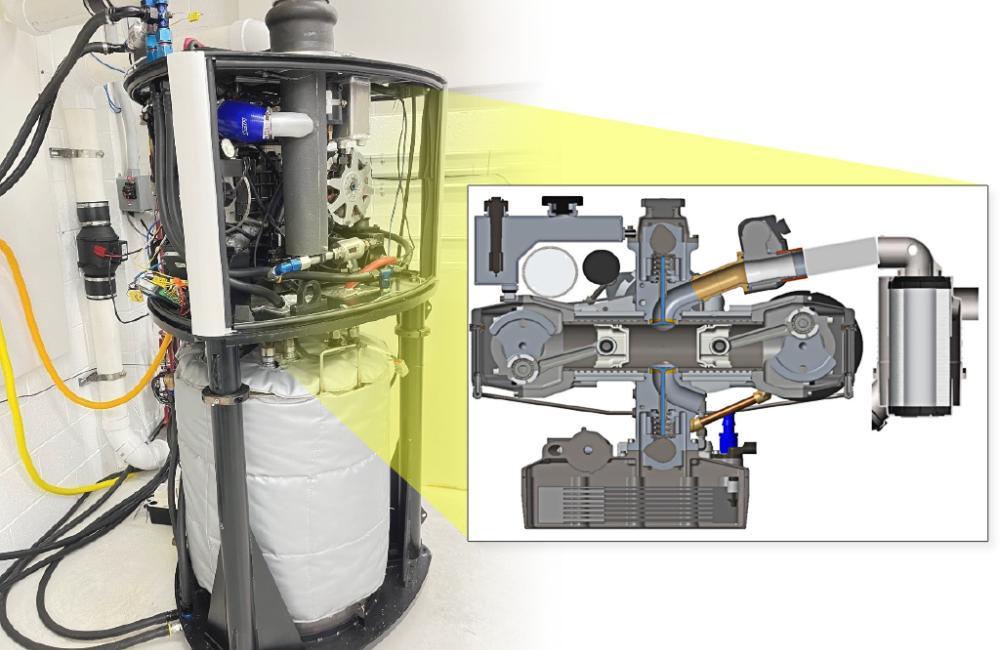
High-Efficiency Micro Combined Heat and Power Device
ORNL and Enginuity Power Systems developed a high-efficiency 8-kilowatt micro-CHP, or combined heat and power device, powered by an opposed-piston engine to simultaneously generate electricity and provide heat to residential or light commercial buildings. The micro-CHP can run on natural gas, renewable biogas and hydrogen, enabling the transition from conventional fossil fuels to zero-carbon fuels.
Residential homes and light commercial buildings require substantial heat and electricity simultaneously. However, two-thirds of the energy used by electricity generation in conventional power plants is wasted in the form of heat discharged to the atmosphere, and additional energy is wasted during the transmission and distribution of electricity to end users. This device efficiently generates electrical power and captures heat that would otherwise be wasted, providing useful thermal energy — hot water used for space heating and hot water supply — in a single process and from a single energy source.
The research was funded by DOE’s Building Technologies Office in the Office of Energy Efficiency and Renewable Energy.
Contributing to the technology were Zhiming Gao, Kashif Nawaz, Ahmad Abuheiba and Brian Fricke of ORNL; and Philip Zoldak, Jacques Beaudry-Losique, Jonathan Mansinger and Maysam Molana from Enginuity Power Systems.
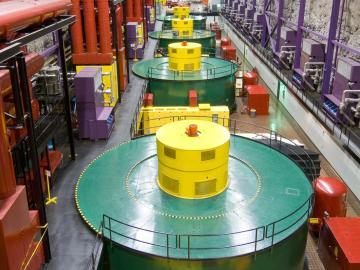
InertiaMeter: Cost-Effective Real-Time Power System Inertia Monitor
Hydropower is a renewable energy source directly connected to the grid, providing inertia as water spins large turbines. Pumped storage hydropower, or PSH, draws electricity from the grid to pump water in times of low power demand to create an energy storage bank. In times of high demand, PSH generates electricity as water is used from the reservoir to turn the turbines.
Scientists at ORNL and the University of Tennessee, Knoxville, developed this algorithm to predict electric grid stability using signals from PSH projects. This provides critical awareness as the grid increasingly shifts to intermittent renewable power. The algorithm calculates inertia using the signal that’s produced when the pumps turn off, combined with information on grid frequency – the system’s overall balance of electricity supply and demand.
The InertiaMeter project also garnered a Silver award in the R&D 100 Special Recognition: Green Tech category.
The project was supported by the DOE's Water Power Technologies Office of in the Office of Energy Efficiency and Renewable Energy.
A team of researchers at the University of Tennessee, led by UT-ORNL Governor’s Chair Yilu Liu and UT’s Hongyu Li and Wenpeng Yu, developed the technology.
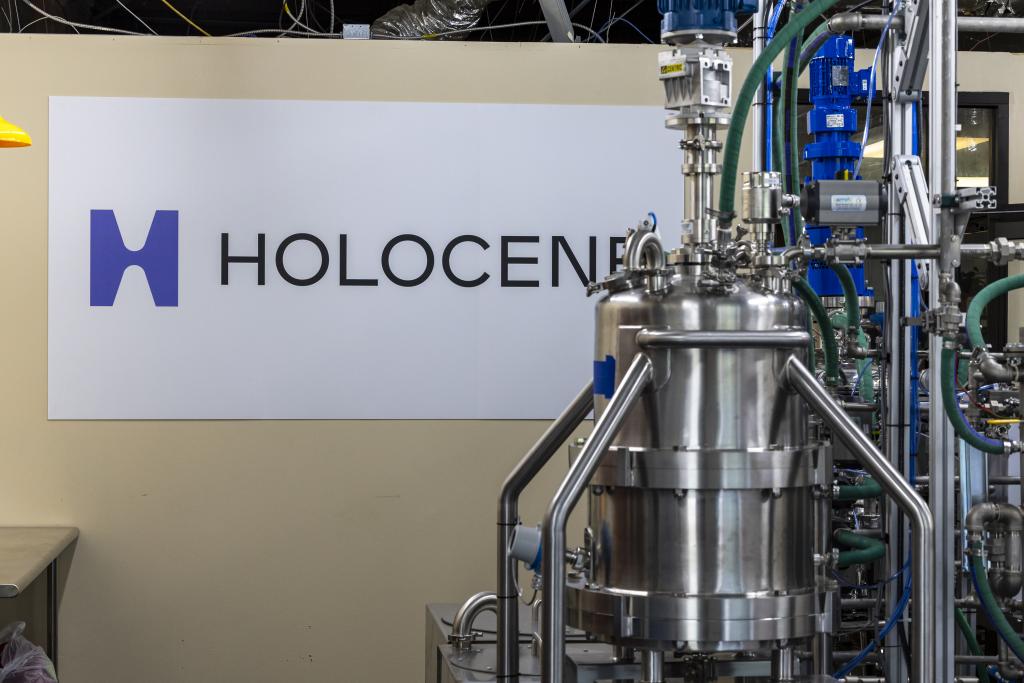
advancing technology from bench scale to pilot scale demonstrations. Credit: ORNL, U.S. Dept. of Energy
APEX CDR – Amino acid Process for EXtreme Carbon Dioxide Removal
An ORNL team developed a direct air capture, or DAC, process developed as a possible solution to global warming. Called APEX CDR, it employs environmentally benign amino acid-based solvents for DAC of carbon dioxide from the atmosphere. This scalable process employing aqueous solvents of amino acid salt solutions, such as potassium sarcosinate, with ethylene or triethylene glycol additives to extract carbon dioxide directly from air, boasts competitive carbon dioxide uptake capacities with relatively low energy requirements at extreme temperatures.
Solvent additives, including ethylene or triethylene glycol, allow the amino acid-based solvents to be effective even at extreme temperatures without freezing. These solvents exhibit competitive energy requirements. The performance of DAC solvents is an area often overlooked despite regions with sub-ambient temperatures comprise a significant portion of the Earth's surface. This process offers significant advancements in efficiency, scalability and economic viability of amino acid-based DAC technologies, paving the way for their widespread implementation in combating climate change.
This research was funded by ORNL Laboratory Directed Research and Development funds through DOE’s Office of Science.
Developing the technology were ORNL scientists Costas Tsouris, Radu Custelcean, Abishek Kasturi, Gyoung Gug Jang, Diāna Stamberga, Aye Meyer, Amiee Jackson, Jonathan Willocks, Scott D. Palko, David S. Sholl; Anca Timofte of Holocene; Jorge Gabitto of Prairie View A&M University; and Dhruba Deka of the Pacific Northwest National Laboratory. Holocene is a Cohort 2022 Innovation Crossroads participant.
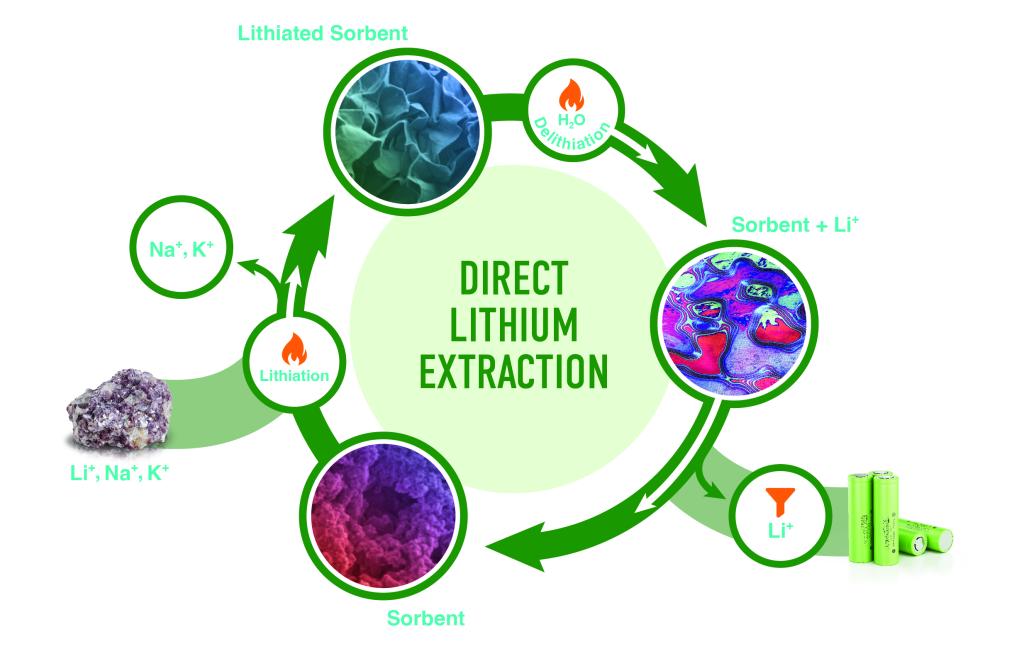
High Lithium Capacity Sorbents for Direct Lithium Extraction Toward Increased Domestic Critical Mineral Supply Chain
Lithium demand is increasing and better ways extracting lithium are needed. This technology, lithium carbonate or lithium hydroxide recovered from brines, clay minerals and recycled lithium-ion batteries, can improve the economics of lithium extraction for energy storage applications. The ORNL team has demonstrated that lanthanum manganese oxide and aluminum oxide are effective sorbents for selective extraction of lithium chloride or lithium sulfate from brines and recycled battery leachates, offering the possibility of effective application of lithium salts in lithium-ion batteries and leading to a fundamental shift in the lithium supply chain.
The main goal of this technology is to develop materials and processing technologies that enable significant new production of lithium from domestic resources by 2025. New lithium production must increase fourfold to meet demand and efficient methods for recovery, concentration and purification must be developed to lower costs, reduce the carbon footprint, conserve water resources and save energy. The ORNL team developed a series of lithium–aluminum-layered double hydroxide (LDH) chlorides (LDH-Cl) and iron-doped LDH, doped lithium manganese oxide and aluminum hydroxide sorbents to selectively extract more than 90% lithium over sodium and potassium in geothermal and oil field brines, clay minerals and recycled lithium-ion battery leachates. With its favorable environmental and economic performance, lithium sorption coupled with solvent extraction has great potential to enable the domestic production of battery lithium compounds.
This research was partly funded by DOE’s Critical Materials Innovation Hub in the Advanced Materials and Manufacturing Technologies Office and ReCell Center, Advanced Battery Recycling, Vehicle Technologies Office and Technology Commercialization Fund, Geothermal Technologies Office in the Office of Energy Efficiency and Renewable Energy.
Developers are Parans Paranthaman, Bruce A. Moyer, Jayanthi Kumar, Ramesh Bhave, Syed Islam, Barbara Evans, Saurabh Prakash Pethe and llja Popovs of ORNL; Bill Bourcier and Chris Camire of Mineral Selective Technologies; Aaron Celestian from the Natural History Museum of Los Angeles County; and Hood Whitson of Element3.
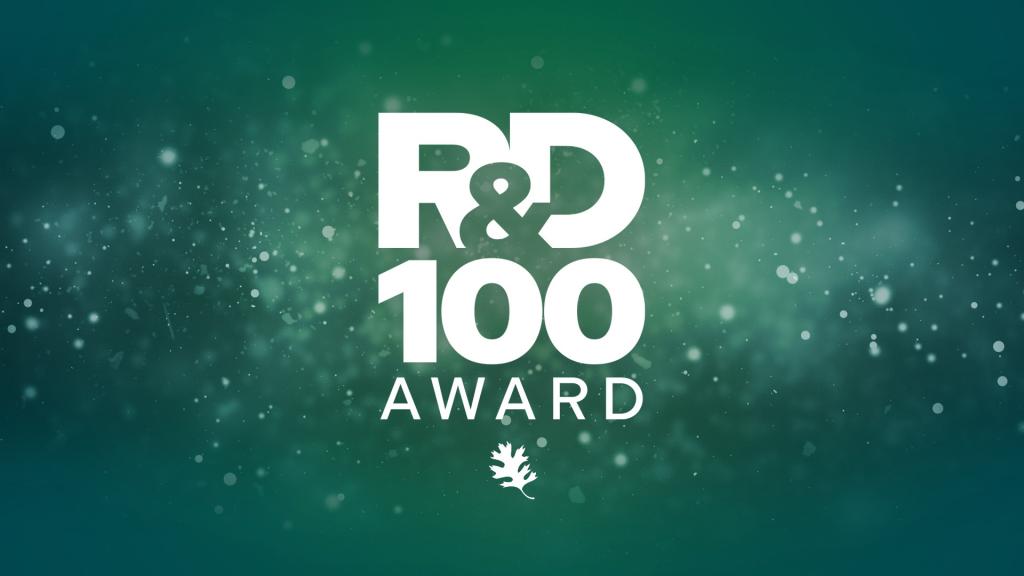
Direct Recycling and Remanufacturing of Li-Ion Battery Electrode Scraps
ORNL scientists have developed a new approach to directly recycle battery scraps using a green solvent. This method efficiently separates electrode materials from metal foils at lower temperatures without impacting their quality. The recovered materials are ready for reuse in new batteries, reducing costs and environmental impact. This direct recycling process can be integrated into existing manufacturing lines with minimal investment, potentially saving more than 10% in production costs and supporting the sustainability of battery manufacturing.
Lithium-ion batteries are essential in electronics, electric vehicles and renewable energy storage, leading to a booming market, particularly for EV batteries. However, battery manufacturing wastes valuable materials such as cobalt, lithium, nickel, copper and graphite, with up to 10% of these cathode materials being discarded. Traditional recycling methods, such as pyrometallurgy and hydrometallurgy, are costly and complex, often breaking down materials too much or requiring the use of harsh chemicals.
The recovered materials can be directly re-manufactured as new electrodes and can be seamlessly reintegrated into fresh batteries through the same production lines while delivering equivalent electrochemical performance. Overall, this new direct recovery and reuse approach is a sustainable process that greatly advances competitiveness of battery manufacturers.
This work was funded by DOE’s Vehicle Technologies Office in the Office of Energy Efficiency and Renewable Energy.
Developers of this technology are Ilias Belharouak, Yaocai Bai, Lu Yu, Ali Abouimrane, and Ruhul Amin of ORNL; and Vivek R. Shinde Patil and Shibani Shinde Patil of Appalachian Renewal Technologies.
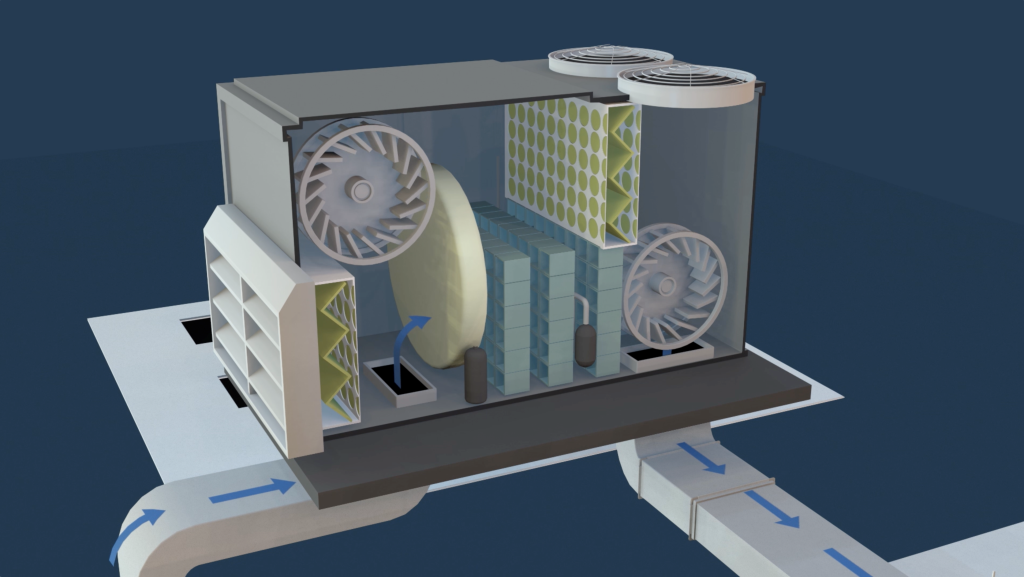
MBE-DAC: Multifunctional Building Equipment for Direct Air Capture
MBE-DAC turns existing rooftop air-conditioning and ventilation equipment into direct air capture, or DAC, platforms to capture carbon dioxide from the air and the building’s ventilation airstreams. The technology collects carbon dioxide directly from air that passes through it by utilizing existing commercial and domestic air-handling systems without adding significant cost and energy consumption. The carbon dioxide is collected on a solid sorbent developed at ORNL and is pure enough to be recycled in chemical processes. The sorbent can also be regenerated and reused. MBE-DAC is designed to be fitted to heating, ventilation and air-conditioning, or HVAC, and other air-handling systems using a modular approach for installation in residential and commercial buildings.
The research was funded by DOE’s Fossil Energy and Carbon Management.
The technology was developed by ORNL staff Kashif Nawaz, Brian Fricke, Costas Tsouris, Michelle Kidder, Chris Janke, Xin Sun, Josh Thompson, Kai Li, Cheng-Min Yang, Steve Kowalski, Keju An, Jamieson Brechtl and Tugba Turnaoglu.
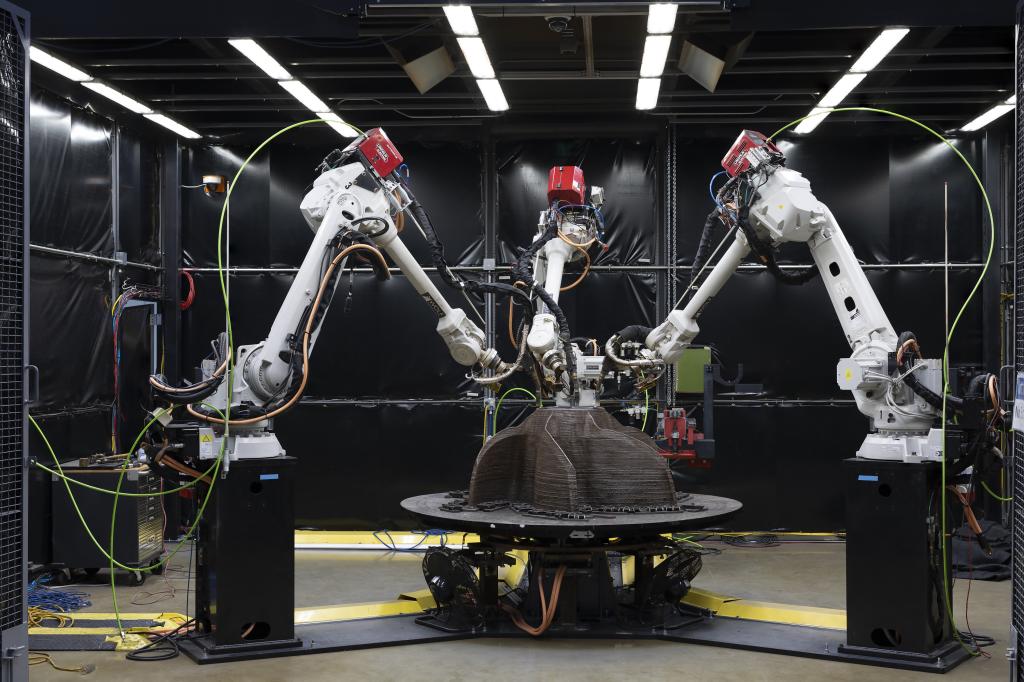
MedUSA: Large-scale Multi-agent Wire Arc Additive Manufacturing
MedUSA is an ORNL-developed multi-agent additive manufacturing system that uses three robotic arms, each equipped with a welder, to collaboratively create near-net-shape parts via wire-arc additive manufacturing.
The welders in MedUSA deposit melted metal layer upon layer to create components in significantly less time than traditional manufacturing techniques and can create complex shapes not achievable through other approaches. MedUSA can produce parts from most metals for which welding wire is available, including a variety of low-alloy steels, stainless steels, tooling steels, Invar and copper- and nickel-based alloys. This means that the system is suited to produce a wide range of end-use components in a less carbon-intensive process than traditional manufacturing methods such as casting or forging.
MedUSA promises to address supply-chain challenges in production of large-scale tooling, which is mostly made overseas and can take years to acquire. The flexibility of the system also means that large parts, which generally are produced in small volumes, can be made more economically. This makes production of components for large-scale clean energy applications, like wind energy, hydropower or next generation nuclear power, significantly more attractive for industry.
The research was funded by DOE’s Advanced Materials and Manufacturing Technologies Office in the Office of Energy Efficiency and Renewable Energy.
Developing the technology were ORNL staff Andrzej Nycz, Alex Arbogast, Chris Masuo, Mark Noakes, Peter Wang, Luke Meyer, William (Bill) Carter, Derek Vaughan, Alex Walters and Joshua Vaughan; and Jonathan Paul and Jason Flamm from Lincoln Electric Additive Solutions.
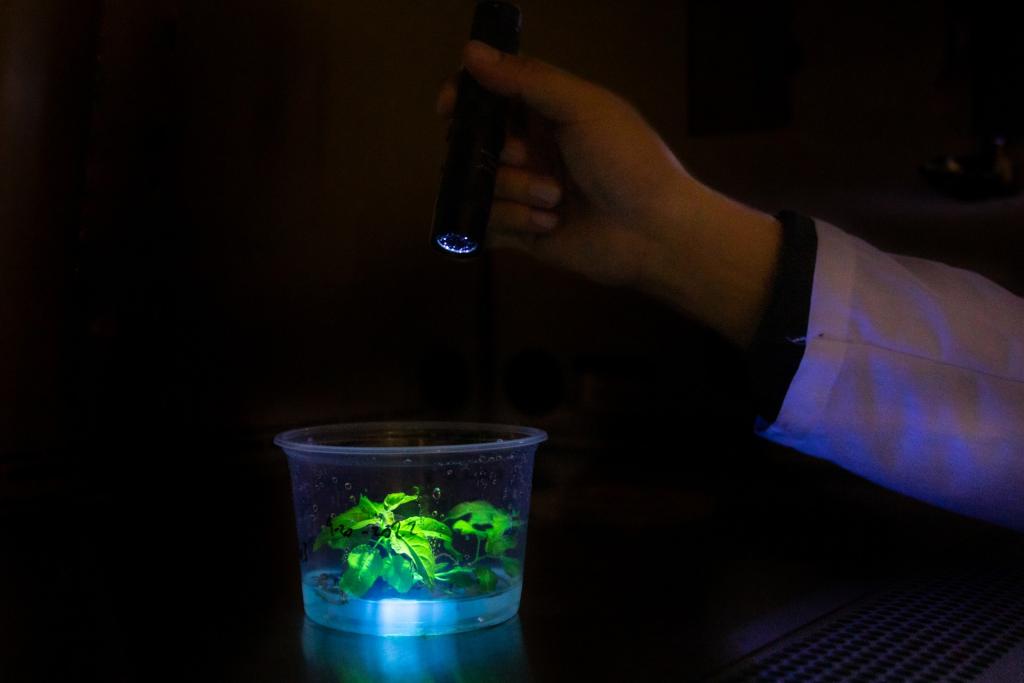
Split-Marker for Gene Stacking
This novel system for genetic engineering in plants makes it easy to add and study multiple genes at once, doubling the speed and halving the cost of plant transformation. This genetic engineering system uses a novel split selectable marker to insert multiple genes into a plant cell simultaneously. This means you only need one marker to select and track the success of adding these genes. It also allows easy identification of transgenic events – identifying which plants have the new genes – using the naked eye through visualization of two reporters: RUBY, which turns the leaf red under white light, and GFPuv, which causes the plant to glow green under UV light.
This technique is particularly adept at several uses: For example, trait stacking, which incorporates multiple desirable traits such as disease resistance or drought tolerance and increased yield into a single crop variety; functional genomics, to study how different genes work together in a plant, providing insights into the functions of genes; pathway engineering, such as modifying metabolic pathways or engineering new pathways by co-expressing multiple genes involved in the same biochemical pathway; and multi-gene editing in which a large number of genes can be modified easily using advanced techniques such as CRISPR/Cas-based systems.
This plant co-transformation system offers a powerful tool for improving plants and understanding their genetic functions with applications for plant science, agriculture, forestry, bioenergy, biomaterials and climate change mitigation.
The Biological and Environmental Research program in DOE’s Office of Science provided funding for this research.
ORNL’s Xiaohan Yang led development with Guoliang Yuan, Gerald Tuskan, Md Mahmudul Hassan and Haiwei Lu, also from ORNL.
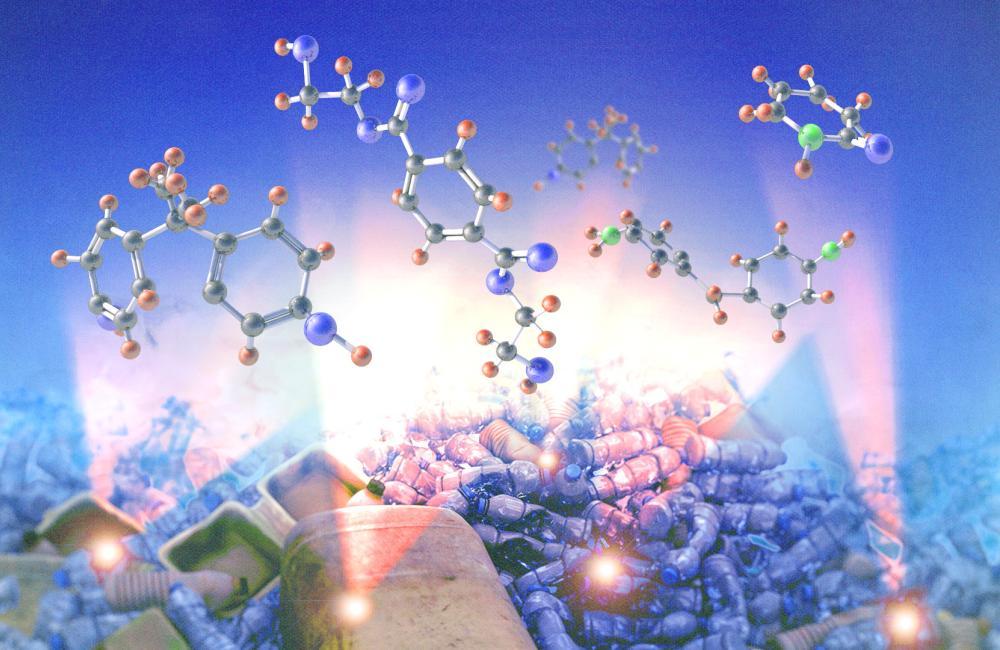
Selective Mixed Plastic Recycling
Less than 10% of plastic waste is recycled. ORNL scientists have developed an extremely effective method that transforms a variety of plastic and mixed plastic wastes into valuable chemicals, thereby significantly decreasing the use of fossil fuels, greenhouse gas emissions and energy consumption.
The novel organocatalyst can deconstruct all kinds of bottles, packaging, foams, lenses, textiles and carpets, which often exist as mixed plastics and are very difficult to recycle by conventional technologies. This method provides a new pathway to produce valuable chemicals from mixed plastic wastes. This technology can transform the field of plastic recycling by adding significant commercial values because inefficiency or inability to convert various plastics and their mixture to valuable materials have been the major bottlenecks in plastic recycling. The technology has various benefits over current recycling technologies. Transforming plastic wastes to useful resources for chemical production by a new technology provides a critical strategy for achieving a decarbonized sustainable society.
This research was funded by the DOE Office of Science and ORNL’s Technology Innovation Program.
The work was done by ORNL staff Tomonori Saito, Md Arifuzzaman now at Re-Du, Bobby Sumpter, Changwoo Do, Zoriana Demchuk, Sheng Dai, Ilja Popovs, Jeff Foster, Nick Galan and Jackie Zheng; and Robert Davis from the University of Virginia. Re-Du is a Cohort 2023 Innovation Crossroads participant.
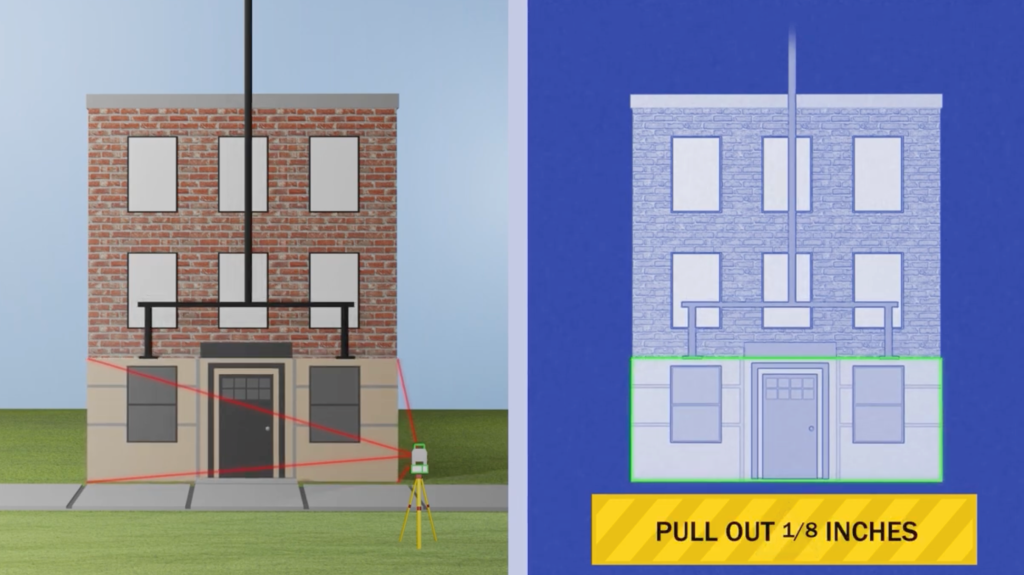
Real-Time Evaluator for Fast and Accurate Installation of Prefab Components
The Real-Time Evaluator, or RTE, improves the process for installing prefabricated building components by providing feedback in real time with the use of advanced software, surveying tools and 3D digital models of the building, called digital twins, to guide the precise placement. The tool can align the digital model with the actual building to within an eighth of an inch accuracy. A robotic station guides installation for the direct placement.
Traditionally, installing large, prefabricated parts such as walls and concrete segments involves manual work and communication between workers and crane operators. The process is imprecise and can lead to costly delays. The RTE provides instant feedback and gives builders immediate instructions through a mobile phone application to show how to adjust placement to meet installation requirements. Real-time feedback constantly updates the digital model to minimize errors and improve accuracy. This technology reduces component assembly time by up to 25% and can be used in new buildings and existing infrastructure.
The research was funded by DOE’s Building Technologies Office in the Office of Energy Efficiency and Renewable Energy.
Developing the technology, all from ORNL, are Diana Hun, Nolan Hayes, Bryan Maldonado, Mengjia Tang, Balaji Selvakumar, Peter Wang, Philip Boudreaux and Stephen Killough.
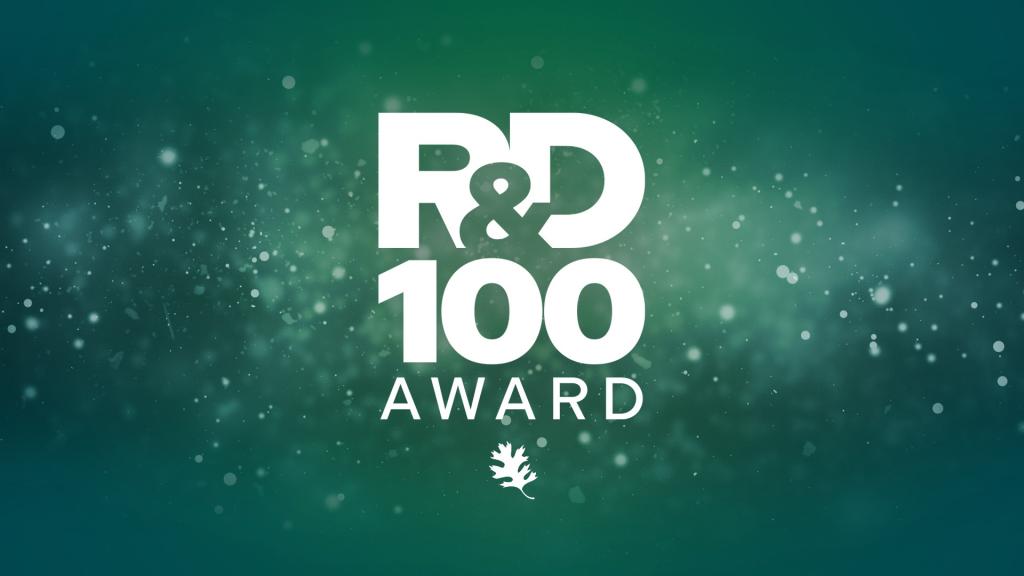
IRIS-SDK: Intelligent Runtime System for Extremely Heterogeneous Computer Architectures
IRIS is a unique runtime system designed to work with various types of computer hardware, boosting performance, portability and productivity. It’s the world’s only framework capable of concurrently executing application tasks on a diverse array of devices simultaneously, including CPUs, NVIDIA GPUs, AMD GPUs, field-programmable gate arrays, or FPGAs, and Hexagon digital signal processors, or DSPs, without any extra work from developers. IRIS is available open source in github. It comes with a software development kit, or SDK, composed of comprehensive suite of tools: Hunter – task scheduling policies hub, Dagger – robust tool validating IRIS runtime and task scheduling policies, and MatRIS – a genuinely heterogeneous and portable linear algebra library, which delivers BLAS, LAPACK and math operations.
IRIS represents a pioneering leap in intelligent runtime systems tailored for extremely heterogeneous computer architectures. It makes programming for diverse computer setups much easier by handling tasks such as heterogeneous kernel execution, memory management, data transfers and scheduling. All this is made automatically and transparently to the users, elevating and taking the programming productivity to a different level. IRIS-SDK enables the application code to write once and deploy everywhere in the heterogeneous computing environment. It improves the performance of application with heterogeneous execution and makes it easy for them to run on both high-performance and embedded systems without needing changes to the code.
IRIS research was funded by the DOE Office of Science.
The development team, all from ORNL, includes Jeffrey S. Vetter, Beau Johnston, Jungwon Kim, Seyong Lee, Narasinga Rao Miniskar, Mohammad Alaul Haque Monil, Pedro Valero-Lara and Aaron Young.
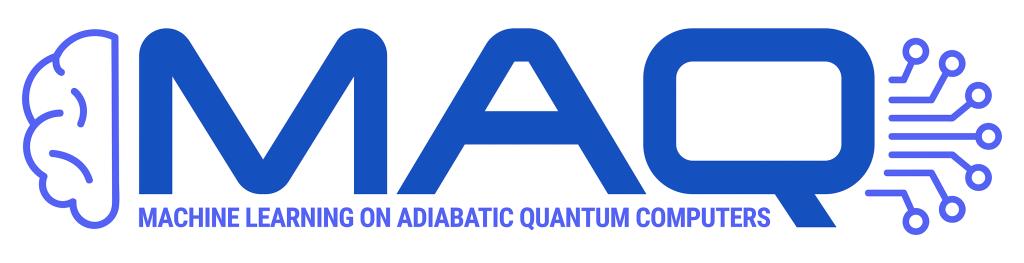
MAQ: Machine Learning on Adiabatic Quantum Computers
ORNL-developed MAQ is the first software that enables developers to leverage the speed of quantum computing to train machine learning algorithms significantly faster than using classical computers without affecting accuracy. Any application, from artificial intelligence to industrial systems, that depends on machine learning now can benefit from faster training leading to rapid deployment.
The MAQ software speeds up the training of machine learning models using a type of quantum computer called an adiabatic quantum computer, or AQC. Quantum computers, especially AQCs, are good at solving complex problems faster. The MAQ library shows that AQCs can train machine learning models much quicker than regular computers, for example, up to five times faster for certain tasks compared to classical methods.
In addition to speeding up training, MAQ includes a special method for embedding problems onto quantum computers, which is more efficient than previous methods. MAQ’s methods could benefit many fields, such as science, business and healthcare, by making data analysis faster and more effective. In fact, the MAQ library methods have a direct impact on industrial and societal applications. Machine learning significantly impacts both industries and society by streamlining operations, enhancing decision-making and improving quality of life. In industry, it boosts efficiency through predictive maintenance, supply chain optimization and personalized services across sectors such as healthcare, retail and manufacturing.
Societally, machine learning advances healthcare diagnostics, personalized education, environmental conservation and public safety. It also supports sustainable agriculture, urban planning and accessibility for people with disabilities. MAQ methods have potential to revolutionize machine learning applications by accelerating the training process.
The research was funded by ORNL's Laboratory Directed Research and Development SEED program through the DOE Office of Science.
The developers are Prasanna Date, Kathleen Hamilton, Robert Patton, Travis Humble and Thomas Potok, all from ORNL.
UnifyFS
ORNL also co-led the UnifyFS project, an R&D 100 Award winner in the Software/Services category. Co-developed by Lawrence Livermore National Laboratory, ORNL’s National Center for Computational Sciences and the University of Illinois, UnifyFS is an evolving user-level file system that makes node-local storage as easy to use as a parallel file system, improving performance and usability for scientific applications.
ORNL’s Sarp Oral and team contributed to the project.
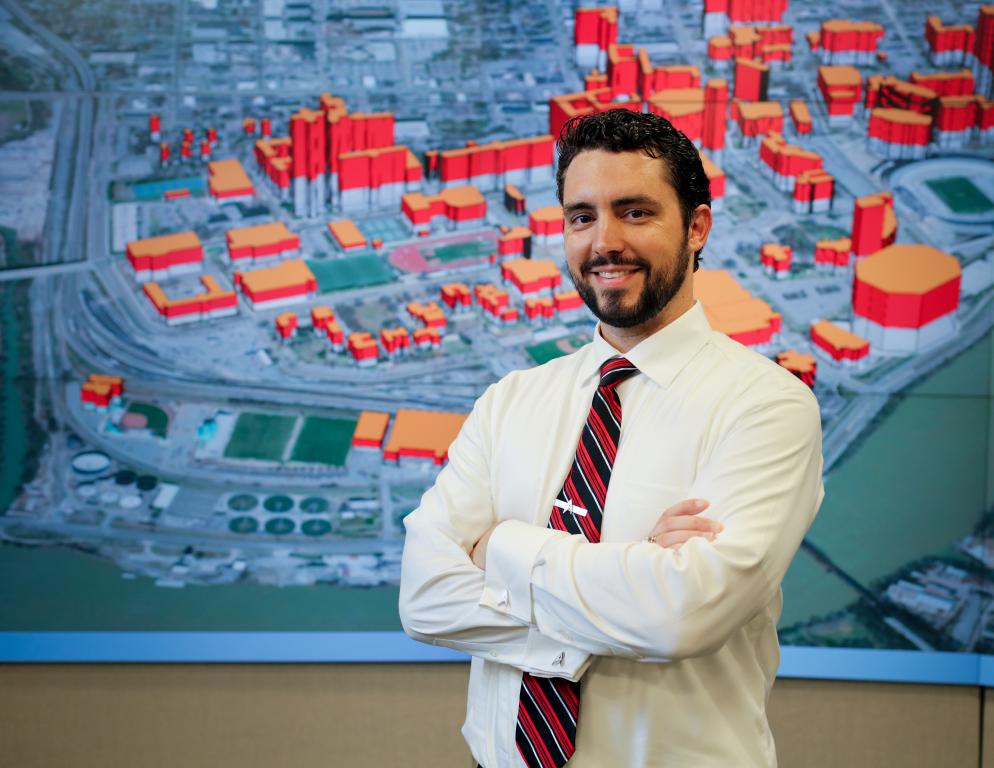
New honored as R&D Researcher of the Year
Joshua New, ORNL Distinguished R&D staff member, won the R&D Researcher of the Year Award. New won for his many contributions to building science, energy efficiency and climate change research. Throughout his career, New has averaged 12.4 publications per year, and had consistent success securing significant research funding, averaging $9 million annually. He also is dedicated to mentoring and knowledge sharing, “ensuring the next generation of researchers are prepared to address future sustainability challenges.”
New developed DOE’s Roof Savings Calculator, an industry web tool for estimating savings from cool roofs, which helped him win a previous R&D 100 Award. He also created the Autotune calibration methodology to make building energy models match measured utility data, which is approved for use in financial decisions. His Automatic Building Energy Modeling, or AutoBEM, software suite led to the creation of energy models for all 125.7 million buildings in the U.S.
To inquire about licensing opportunities related to these technologies, please contact partnerships@ornl.gov.
UT-Battelle manages ORNL for the Department of Energy’s Office of Science, the single largest supporter of basic research in the physical sciences in the United States. The Office of Science is working to address some of the most pressing challenges of our time. For more information, please visit energy.gov/science. — Lawrence Bernard