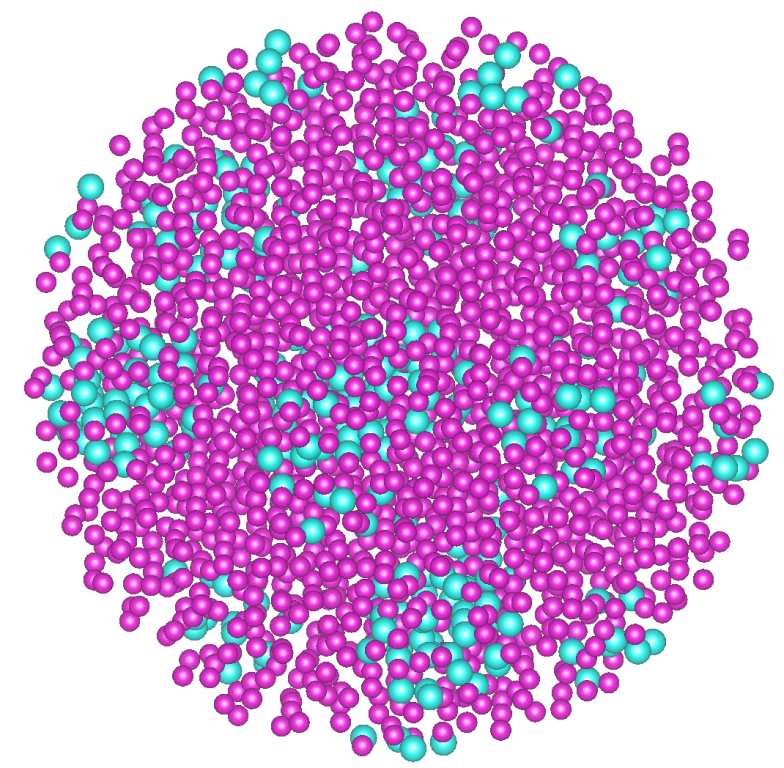
Researchers used the world’s first exascale supercomputer to run one of the largest simulations of an alloy ever and achieve near-quantum accuracy.
The study led by the University of Michigan’s Vikram Gavini employed Frontier, the 1.14-exaflop HPE Cray EX supercomputer at the Department of Energy’s Oak Ridge National Laboratory, to take a first-principles approach to simulation via the Schrödinger equation, which describes the evolution of microscopic systems, including their probabilistic nature. Results could be used to help design candidates for new alloys and to fuel other computational design efforts such as drug discovery.
The work received a finalist nomination for the Association of Computing Machinery’s 2023 Gordon Bell Prize, which honors outstanding achievements in high-performance computing. The prize will be presented at this year’s International Conference for High Performance Computing, Networking, Storage and Analysis, or SC23, in Denver where the team will present their results on Nov. 14.
“The promise of our study shows this kind of approach should be widely useful across many areas of science and answer some of the challenging questions that have persisted for decades, from aerospace to medicine,” Gavini said.
Gavini’s team used an integrated computational framework on the Frontier and Summit supercomputers, both housed at ORNL’s Oak Ridge Leadership Computing Facility, to simulate dislocations, or defects, in a magnesium system of nearly 75,000 atoms. Magnesium alloys make promising candidates for lighter alloys, but the lack of dislocations in magnesium’s atomic structure can lead to brittleness and cracking. Understanding dislocations in magnesium alloys could lead to lighter, more flexible alloys for industry.
Separately, the team used the National Energy Research Scientific Computing Center’s Perlmutter supercomputer to study the stability of quasicrystals — a structure that’s ordered but not periodic — in an ytterbium-cadmium alloy.
The calculations relied on density functional theory, a quantum-mechanical approach to computing materials’ atomic and electronic structure, and used machine learning to achieve a level of accuracy close to that offered by quantum many-body calculations. The largest calculation reached 659.7 petaflops using 8,000 nodes of Frontier.
“As we push to achieve greater accuracy, the accessible system sizes of the calculations dramatically drop,” Gavini said. “We took the results of quantum many-body calculations on smaller systems and used machine learning to infer a universal constitutive relationship of electrons that could be used in larger density functional theory calculations. Marrying these approaches allows us to keep the advantage of a large machine like Frontier while approaching quantum accuracy.”
The study marks the latest milestone in a decade-long effort by Gavini and his team. A 2019 study that used Summit to simulate more than 10,000 magnesium atoms also earned a Gordon Bell Prize nomination.
Production of alloys relies on melting metals, mixing them and then pouring the liquid into molds to harden. During solidification, defects form that can either aid or be detrimental to material properties. The atomic structure of a material plays a crucial role in the behavior of these line defects, often called dislocations.
Ductile metals like aluminum benefit from an atomic structure that allows the metal to accommodate dislocations and their motion. Magnesium’s atomic structure fails to accommodate dislocations as easily, making it more brittle.
“These kinds of defects can create unprecedented properties under the right circumstances,” Gavini said. “Why do these defects form? How can we use these defects to bring about desired rather than undesirable properties? In the previous study, we looked at the energy of a single dislocation in bulk magnesium. In this study, we looked at interacting extended defects in a magnesium alloy.”
Results yielded the most detailed picture yet of such a structure, at an accuracy approaching quantum accuracy. Gavini hopes to apply the methods to a wide range of studies.
“If we can do these large-scale calculations at near-quantum accuracy, that means we can use these calculations to help design better materials with computational design, to explore compounds for drug discovery, to understand properties of nanoparticles and material systems at new levels of detail,” he said. “Without exascale and Frontier, we wouldn’t be able to do these types of calculations. Now that we know how to do them, we can apply these methods broadly to explore other questions.”
Support for this research came from the Exascale Computing Project, a collaborative effort of the DOE Office of Science and the National Nuclear Security Administration, the DOE Basic Energy Sciences program, and the DOE Office of Science’s Advanced Scientific Computing Research program. The OLCF is a DOE Office of Science user facility.
UT-Battelle manages ORNL for DOE’s Office of Science, the single largest supporter of basic research in the physical sciences in the United States. DOE’s Office of Science is working to address some of the most pressing challenges of our time. For more information, visit https://energy.gov/science. – Matt Lakin