75 years of science and technology
Oak Ridge National Laboratory today is an open science laboratory that attracts thousands of scientists and engineers from around the globe each year. It began in the World War II Manhattan Project, which developed the first atomic bombs.
In summer 1939, tensions were rising among European powers. Nazi Germany invaded Czechoslovakia in March. Weeks later, imperialist Italy invaded Albania. Across the Atlantic, U.S. President Franklin Roosevelt struggled with America’s neutrality policies, which prevented him from providing arms to Britain.
Meanwhile, outside the world of politics, in the halls of universities and research institutes, physicists were reeling from an amazing discovery.
The age of the atom
Scientists’ understanding of the atom had rapidly taken shape in the first decades of the 20th century. Following the discovery of radioactivity by French physicist Henri Becquerel in 1896, British physicist Ernest Rutherford theorized that the phenomenon was caused by the breakdown of atoms. In 1913, Danish physicist Niels Bohr applied Rutherford’s work to the relatively new theories of quantum mechanics to develop the first model of the atom with electrons surrounding the nucleus in shells.
The discovery of protons, and later neutrons, allowed scientists to assign each element an atomic number, based on the number of protons in the nucleus, and to explain the existence of isotopes, based on the number of neutrons.
By the 1930s, scientists including E.O. Lawrence at the University of California and Robert Van de Graaff at Princeton University were using new instruments called particle accelerators to study the properties of nuclei by striking them with high-energy protons. Italian physicist Enrico Fermi used neutrons instead of protons—taking advantage of the neutral particle’s ability to probe the nucleus without resistance—and he produced a slew of new radioactive isotopes.
The nucleus seemed to hold limitless possibilities.
Then, in December 1938, German chemists Otto Hahn and Fritz Strassmann discovered something unusual when they bombarded uranium with neutrons: The experiment produced radioactive isotopes of the lighter element barium.
Hahn described this puzzling result in a letter to his former colleague, Austrian physicist Lise Meitner, who had fled Nazi Germany for Sweden in July 1938. Meitner and her nephew, physicist Otto Frisch, discussed the problem and concluded that the neutron had split the uranium atom into lighter atoms and converted some of its mass to energy, in agreement with Albert Einstein’s famous equation “E = mc2.” Frisch discussed their findings with Bohr, who announced the discovery of fission at a conference in St. Louis in January 1939.
Named after the technical term for cell division—binary fission—the nuclear phenomenon would become the subject of a flurry of experiments and academic papers in Europe and the United States over the next few months.
A chain reaction begins
While physics laboratories across the United States repeated and advanced the German discovery of fission, three physicists in particular worried how this new knowledge would influence political and military powers.
The discovery unlocked the possibility of a chain reaction in which neutrons ejected from one split atom would collide with new atoms, leading to a series of fissions. With each split, more energy would be released. “Criticality” would be achieved when the process reached a critical tipping point and continued unabated.
Hungarian-American physicists Leo Szilard, Eugene Wigner and Edward Teller feared Germany would obtain enough uranium to weaponize nuclear fission. They also realized that this discovery was not known or understood outside the scientific community. To help elevate their concerns to the government, Szilard rushed to meet with his acquaintance Einstein, who had become quite famous since moving to the United States in 1933.
Interrupting Einstein’s vacation at a beach house on Long Island, Szilard and Wigner persuaded the popular genius to write a letter to the Belgian ambassador to the United States (because Belgium controlled uranium resources in the Congo), explaining the nuclear potential of the metal. As Wigner later recalled, “Einstein understood it in half a minute. It was really uncanny how he dictated a letter in German with enormous readiness.” Wigner translated the letter to English.
Then a mutual friend of Szilard and President Roosevelt, the economist Alexander Sachs, offered to deliver a letter directly to the White House. In that letter, Einstein implored the president to “have some permanent contact maintained between the Administration and the group of physicists working on chain reactions in America.” He ended the letter by warning that Germany had stopped the sale of uranium from the Czechoslovakian mines it had just seized, leading to an unnerving speculation that Germany was on the path to “extremely powerful bombs of a new type.”
It was two months before Sachs met with Roosevelt and shared the letter, but the president took immediate interest. “What you are after is to see that the Nazis don’t blow us up,” Roosevelt told Sachs.
Within a week, Roosevelt set up an Advisory Committee on Uranium. What would transpire over the next few years would be the largest collaboration between the scientific community and government in U.S. history.
Three paths to atomic weapons
While nuclear research continued at universities from 1939 to 1941, the federal government slowly organized its response to the uranium problem. Roosevelt’s uranium committee evolved into the Section on Uranium, or S-1, of the Office of Scientific Research and Development, a new government office with authority to fund scientific missions.
After the December 7, 1941, attack on Pearl Harbor drove the United States to declare war, S-1 accelerated research on pathways for developing what had come to be called an “atomic” bomb. The biggest hurdle would be amassing enough fissionable material to generate a sufficiently powerful nuclear chain reaction.
Fissionable material is rare in nature. Less than 1 percent of natural uranium is the fissionable uranium-235 isotope; most natural uranium is non-fissile uranium-238. One of the first initiatives of U.S. physicists was to prove that it would be impossible to achieve a chain reaction using natural uranium alone. Indeed, it was impossible—which was good (because it would be difficult for Germany to develop a nuclear weapon) and bad (because it would also be more difficult and expensive for the United States to develop one first).
Because natural uranium alone would not work, physicists needed to increase the concentration of fissionable material— a process that would come to be known as enrichment—to produce enough material for an atomic bomb, as well as any peacetime application of nuclear energy.
At the end of 1941, two fissionable materials were under consideration—uranium-235 and an isotope of the recently discovered element plutonium, plutonium-239—as well as three enrichment processes. To enrich uranium-235, researchers proposed processes known as gaseous diffusion and electromagnetic separation. To extract plutonium, researchers proposed a nuclear reactor “pile” in which natural uranium bombarded by neutrons from fissile uranium-235 could be converted, in small amounts, to plutonium-239. The plutonium would then be chemically separated from the other fission products—a process that would prove just as challenging and critical to the project’s mission as building the reactor.
By summer 1942, six months into America’s involvement in the war, the task of building plants to produce uranium-235 and plutonium-239 was assigned to the U.S. Army Corp of Engineers. The Army created the top-secret Manhattan Project—named for the project’s original office in New York City.
The first order of business for the Manhattan Project’s newly appointed director, Gen. Leslie Groves, was acquiring land for the uranium enrichment plants and a pilot plutonium production facility. The Clinch River Valley in East Tennessee met the project’s strategic demands. The valley was far enough inland to discourage enemy attack and remote enough to avoid attention, yet close to the nearby workforce in Knoxville. It was also equipped with abundant electrical power from the Tennessee Valley Authority and cooling water from the nearby Clinch River.
Three secret sites
Across the country Manhattan Project sites in Washington and New Mexico would carry out full-scale plutonium production and weapon development, respectively.
Code-named “Site X,” the 59,000 acres in Tennessee were roughly quartered for three production plants and a townsite to house workers. To the west, the K-25 plant for enriching uranium through gaseous diffusion separation would soon be home to the largest building in the world, containing more than 1.6 million square feet of floor space. (The much smaller S-50 plant, which used a thermal diffusion process for uranium enrichment, would be constructed near K-25 in 1944.) To the east, the Y-12 plant would house calutrons for enriching uranium through electromagnetic separation.
And to the south, X-10 would host the world’s first permanent nuclear reactor and plutonium processing plant, which would serve as a prototype and training facility for larger production plants at the Manhattan Project site in Hanford, Washington.
X-10—the future ORNL—would also enable critical research on plutonium at Los Alamos Laboratory in New Mexico. Plutonium was not discovered until 1940, and no one fully understood how it might react in a fission reaction. But physicists did know that plutonium-239 was about 1.7 times more fissionable than uranium-235 and could not be overlooked. The physicists who would develop the bomb at the Los Alamos site needed samples of plutonium to study—the sooner, the better.
Practically overnight, X-10 became a training ground for hundreds of workers, including many chemists and engineers, who were developing the fields of nuclear engineering and isotope separation.
The world’s first permanent reactor
DuPont, the industrial contractor for X-10 and the full-scale reactors at Hanford, broke ground near Bethel Valley Road on February 2, 1943. The X-10 reactor was built in just nine months and reached criticality—that is, a self-sustaining fission reaction—on November 4 that year.
Named for the lightweight element used to moderate, or slow, neutrons released during fission, the Graphite Reactor was the world’s first continuously operating nuclear reactor. It had infrastructure including controls, ventilation and shielding to support a megawatt of power (and eventually 4 megawatts).
Future ORNL leaders Eugene Wigner and Alvin Weinberg contributed to the design. In particular, Weinberg, as a young researcher on Wigner’s team at the Chicago Metallurgical Laboratory, calculated the layout of the graphite and uranium lattice. Wigner campaigned for the Graphite Reactor to be water-cooled, which he correctly predicted as the preferred design for future nuclear reactors (air cooling would not suffice at higher power outputs), but the Army decided to keep it air-cooled for two main reasons: First, it would be quicker to build an air-cooled reactor, and the scientists at Los Alamos in charge of developing the plutonium bomb needed samples of plutonium to study as soon as possible. Second, the air-cooled reactor could be used to study corrosion in materials for water-cooled reactors, like those being developed at Hanford.
In the Graphite Reactor, small amounts of natural uranium (primarily uranium-238) were converted to plutonium-239 through irradiation. Operators loaded uranium “slugs”—4-inch cylindrical pellets encased in aluminum—into some of the 1,248 channels in the 24-foot cube of graphite. The configuration of uranium and graphite helped the reactor operators predict when the reactor would reach “criticality.” The reaction was further controlled through the use of seven control rods of heavy elements, including boron and cadmium, that absorbed neutrons.
The reactor was also designed to support research. Empty chambers in the graphite block enabled scientists to test materials exposed to radiation, and a 6-foot thermal column of graphite at the top of the reactor provided a strong source of thermal neutrons. Research at the reactor initially focused on testing how radiation affected materials to be used at Hanford and measuring fission products and their half-lives, but its usefulness as a research tool led to many experiments in materials, biology, chemistry and more.
Ultimately, the Graphite Reactor would outlive its wartime purpose, operating for exactly 20 years and enabling many scientific firsts after producing the first gram-sized quantities of plutonium for the war effort. During its lifetime, it was also the world’s foremost source of radioisotopes for medical, industrial, and research applications.
Processing plutonium
As operators inserted new uranium slugs into reactor channels, irradiated slugs were pushed into a trench of water, known as the canal, behind the graphite block. Immersed in water to block radiation, the slugs, now containing traces of plutonium-239, could be transported in buckets via the canal to a chemical processing facility next door, which consisted of six hot cells for plutonium separation.
Extracting plutonium-239 from the uranium mix was a problem not of nuclear science but of chemistry. The first scientist to produce plutonium, American physicist Glenn Seaborg, demonstrated possible methods for separating plutonium-239 at large scales at Chicago’s Metallurgical Lab. Seaborg tested a lanthanum fluoride carrier—a rare earth compound that helped scientists tease out trace amounts of plutonium during chemical separation. Bismuth phosphate, a compound with less corrosive properties, was ultimately chosen for pilot production at X-10 based on the work of Seaborg’s colleague Stanley G. Thompson.
While Metallurgical Lab scientists worked relatively freely with trace levels of these materials in an open environment, X-10 scientists worked with greater quantities of plutonium and thus had to contend with manipulating lab equipment through several feet of radiation-shielding concrete. Yet X-10 chemists managed to advance techniques for handling nuclear materials and improve the chemical separation process so that, by 1944, they could recover about 90 percent of plutonium‑239 found in the uranium solution.
Hundreds of chemists and engineers trained at X-10 soon would take their expertise to Hanford, as plutonium production began at even larger scales in 1944. Developing the equipment and methods for plutonium separation and writing the first scientific textbooks and training manuals for nuclear work were major Oak Ridge contributions to the war effort.
The secret city
Although the East Tennessee valleys chosen for the Manhattan Project production plants were appealing for their relative isolation, they were not vacant. After a 1798 treaty between the U.S. government and regional Cherokee tribes, settlers acquiring land grants had established the rural communities of Elza, Robertsville, Scarboro and Wheat. Through the 19th century, the communities grew to become home to hundreds of people who made their living through farming, milling and mining. The land was dotted with churches, general stores, schools and a liberal arts college.
Today, as employees drive into ORNL from the east, they pass the remaining structures of the Scarboro community, including Scarborough Elementary School (using the former spelling of Scarboro) that’s now part of the Oak Ridge Institute for Science and Education, and New Bethel Baptist Church and the church cemetery en route to ORNL’s main campus.
When the Army filed a declaration in federal court in 1942 to reclaim the land and compensate residents, the families of the Clinch River Valley were given up to 90 days (but often less) to pack up and abandon their homes. As they arrived in nearby towns like Clinton with their possessions strapped on trucks, their exodus signaled the first wave of change.
At home in Oak Ridge
Although the Army led the Manhattan Project, Groves and other leaders recognized that the community must be a compromise between a controlled military installation and the “normal” civilian communities to which scientists and skilled laborers were accustomed.
The Army hired the national architectural firm Skidmore, Owings & Merrill to design a townsite that would provide essentials and a few modest luxuries, including two- and three-bedroom houses for families (complete with fireplaces and porches), a town center with a grocer and other commercial retailers, schools and recreational facilities.
Other residences included dormitories for unmarried or temporary workers, many of whom ended up sharing close quarters as more beds were needed. There were also trailer camps for construction workers and barracks for military personnel, including some onsite at the plants rather than in town.
Services such as a bus system, sewage and garbage disposal, utilities, schools and a hospital were managed by the Roane-Anderson Company. At the height of the project, the company oversaw the ninth-largest bus system in the United States, 17 cafeterias and a hospital with more than 300 beds.
Although the town design surpassed some expectations— the Army was particularly pleased with the prefab housing built with cemesto (a mix of cement and asbestos) that met cost and design requirements—Oak Ridge would be plagued with logistical issues during the war, particularly the never-ending demand for more housing.
In early 1943, the Army estimated Oak Ridge would grow to about 13,000 people, for whom a small number of stores would suffice at “Townsite,” later named Jackson Square. By the fall of 1943, however, the estimate increased to 42,000 people and would rise again in early 1945 to 66,000.
Ultimately, 75,000 people would live in Oak Ridge at the height of operations in summer 1945. More residences were built throughout the war, and five more neighborhood centers appeared. The city went from nine commercial businesses in 1943 to 165 businesses in 1945.
The population boom greatly affected residents who were moved to makeshift housing, including all African-American residents. Initially, a neighborhood of cemesto houses was planned for African-American workers, but as housing demands increased, these homes were rented to white workers, and “hutments”—one-room plywood huts with four to five beds and a stove—were rented to African-American residents, as well as to some white residents working temporary or labor jobs.
Because wages at the plants in Oak Ridge could be about twice the pay in the surrounding area, many African-American workers came to live and work in the Secret City. Their experience was not the same as most employees, however. Oak Ridge was located in the segregated South of the 1940s, and the town was also segregated, with separate public facilities for African-Americans, such as cafeterias and recreation halls. African-American spouses lived in separate housing and were not permitted to bring their children to Oak Ridge until 1945. However, gradual change came after the war and, in 1955, the city became the first in the South with an integrated high school.
‘Let it stay here’
“You are now a resident of Oak Ridge, situated within a restricted military area. ... What you do here, What you see here, What you hear here, let it stay here.”
—Orientation to Oak Ridge, from City Behind a Fence
As soon as plant construction began in February 1943, the Army began staffing its “Safety Forces.” By 1945, Safety Forces grew to include about 750 military police, more than 400 civilian law enforcement officers, and about 4,900 civilian guards. Oak Ridge residents outnumbered these forces by only about 12 to 1.
One of the most common interactions among residents, plant employees and security officers happened during identification and vehicle checks at gated entrances to the town and plant sites. Badge and identification checks were also common within the townsite; even children 12 and older had badges.
Civilian law enforcement also patrolled the commercial centers and residential neighborhoods. Even if no Safety Force personnel were in sight, bulletins and billboards cautioned residents to remain vigilant in their work—and silent.
Resident directories, copies of the town newspaper "The Oak Ridge Journal" and photographs were not allowed outside the barbed wire fences. Some residents were even recruited to mail in reports on suspicious activities they witnessed at work or around town.
But despite the secrecy—and in some ways, because of it—Oak Ridge would become a permanent home for some residents. The town brought together people from across the country who shared the same sense of purpose and lived through truly unique circumstances.
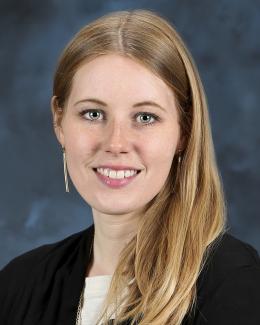