Imagine back nearly 14 billion years. The universe was very small, very hot and very dense. Then it exploded in the Big Bang, quickly producing the protons and neutrons that would eventually constitute nearly all matter in the galaxies and planets we know.
For the first tiny fraction of a second, however, matter was in a form that we almost never see, a form that we didn’t even postulate until the 1960s. Before about a millionth of a second after the Big Bang, the universe was a soup of tiny particles known as quarks and their force carriers, known as gluons.
Quarks and gluons are still around, but we don’t observe them because they hate to be alone. By the rules of the strong nuclear force, they always combine into protons, neutrons and related particles.
“This feature of quarks and gluons, that they’re particles but you can’t observe them as free particles, is something that scientists have tried to understand for a long time,” said ORNL physicist Tom Cormier. “It’s not even fully understood theoretically how that comes about, how something can be hidden like that and rigorously turn back into an ordinary particle before you see it.”
A direct study of quarks and gluons would be very useful to anyone interested in matter and its origins, but such a study requires researchers to re-create that soup of quarks and gluons—technically known as a plasma. That is the job of the international ALICE experiment at the Large Hadron Collider, located along the border of France and Switzerland.
Cormier leads the American contribution to ALICE, which produces quark–gluon plasmas by accelerating lead nuclei along a 17-mile tunnel and smashing them together at essentially the speed of light. The resulting collisions create temperatures 100,000 times hotter than the core of the sun and, for a brief instant, quark–gluon plasmas.
The plasmas themselves are very, very dense, but some of the quarks and gluons—known collectively as “partons”—escape and head toward the surrounding detectors.
They don’t arrive as quarks and gluons. Rather, the fastest-moving escapees create jets of more mundane particles—jets rather than single particles because they carry an enormous amount of energy. It is these secondary particles that travel through chambers filled with ionized, electrified gas, leave detectable tracks, and reach other detectors beyond.
Even though the detectors don’t see quarks and gluons directly, ALICE researchers have gained an unprecedented understanding of the quark– gluon plasma through the analysis of these other particles, a process that has been likened to re-creating the workings of Swiss watches by smashing them together at the speed of light and examining the leftover pieces.
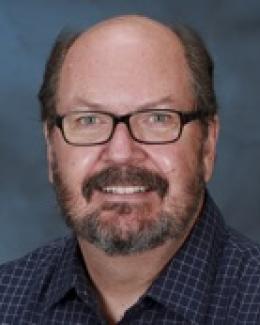